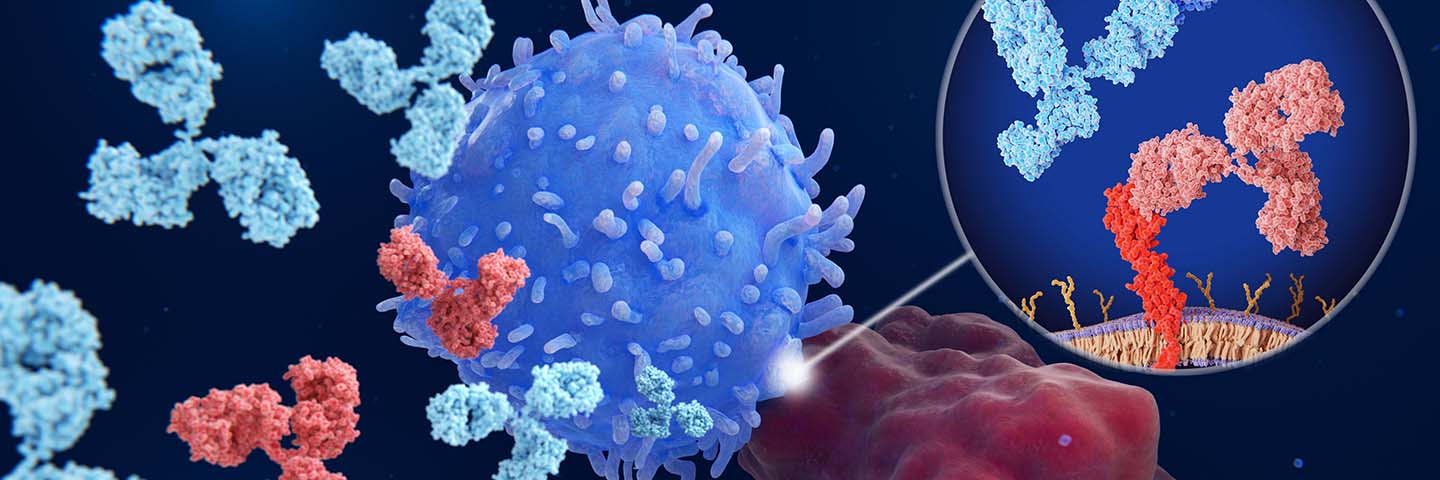
Authors: Hyeyoun Chang, PhD; Kevin Tyo, PhD; John Foulke, MS; Luping Chen, BS; Fang Tian, PhD;* Zhizhan Gu, PhD*
Immuno-Oncology Group, Cell Biology R&D, ATCC, Gaithersburg MD 20877
This study demonstrates the use of three robust, responsive, and reproducible GAS-Luc2 reporter solid tumor cell lines for the assessment of T cell and other immune/tumor microenvironmental cell-mediated immune responses triggered by PD-1/PD-L1, CD-155/TIGIT, or B7-H3 (CD276) immune checkpoints.
Abstract
Introduction
Results with Materials & Methods
Conclusions
References
Abstract
Cancer immunotherapy targeting immune checkpoints, such as immune checkpoint inhibitors (ICIs), antibody-dependent cellular cytotoxicity (ADCC), and antibody-drug conjugates (ADCs), have shown tremendous success in the treatment of solid tumors including skin, lung, breast, renal, and liver cancers. However, the built-in complexity of immunological models and the variable drug responses among different cancer types have been challenging the development and application of these novel immunotherapies. To facilitate large-scale drug discovery for this growing class of immunomodulators, we conducted a comprehensive cell surface protein profiling of ATCC’s vast portfolio of human tumor and immune cell lines for established and novel immune checkpoint molecules, as well as their binding ligands. Based on this protein profiling data, we generated three immune checkpoint reporter cell lines, HCC827-GAS-Luc2, MG-63-GAS-Luc2, and NCI-H1650-GAS-Luc2, that endogenously express high levels of programmed death-ligand 1 (PD-L1), cluster of differentiation 155 (CD155), and B7 homolog 3 protein (B7-H3/CD276), respectively. These reporter cell lines were engineered to contain a gamma interferon activation site (GAS)–response element upstream of a luciferase gene. The luciferase expression is suppressed when the relevant immune checkpoint marker on the cancer cells binds to the corresponding checkpoint protein on T cells. In the presence of the relevant immune checkpoint inhibitor, the GAS-Luc2 reporter cell senses the IFN-γ from the activated T cells to produce a luciferase expression–based bioluminescent signal, which can be readily detected and quantified to evaluate the efficacy, potency, and dynamics of the checkpoint inhibitor. In addition to drug screening for immune checkpoint inhibitors, these GAS-Luc2 reporter tumor cell lines are also proven to be effective in detecting paracrine IFN-γ signaling for immune checkpoint targeted ADCC drug screening.
Download a PDF of this application note
Download nowIntroduction
The success of immune checkpoint inhibitors in the treatment of various types of cancers and their continued growth in the market has driven burgeoning interests in developing more immuno-oncology drugs in this category.1 However, the intrinsic complexity of immunological models and the variable drug responses among different cancer types have challenged this endeavor.2 While immuno-oncology studies have made significant progress by using syngeneic mouse models or humanized mouse models, the inevitable differences between the mouse immune system, or a hybrid human/mouse immune system, and the human immune system remain a concern.3 Hence, interests regarding in vitro fully human immuno-oncology assays have rapidly increased in recent years, many focusing on co-culture assays utilizing various primary human immune cells, especially CD8+ cytotoxic T cells, that take part in killing human cancer cells in vivo.4,5 Nonetheless, the prevailing assays in this category largely employ an artificial system with engineered ectopic overexpression of immune checkpoint molecules and a T cell activation signaling reporter gene in immortalized T cells, such as an engineered Jurkat T cell line (CD4+, non-cytotoxic) with stable expression of human PD-1 and a nuclear factor of activated T cells (NFAT)–driven luciferase reporter.6 More physiologically relevant immuno-oncology reporter assays are urgently needed for better modeling of the in vivo CD8+ cytotoxic T cell–mediated cancer killing events and for immuno-oncology drug discovery and development.
Cancer immunotherapy has been an effective therapeutic approach in many hematopoietic malignancies, especially for the PD1/PD-L1 and CTLA-4 blockade therapies.7,8 Additionally, immune checkpoint blockade therapies for CD-155/TIGIT, as well as targeting B7-H3 have also been investigated and have shown promise in use as immunotherapies.9-12 However, the success rate of these therapies is significantly lower in solid tumors.13,14 Recent studies have demonstrated—both in vitro and in vivo—that the interferon-γ receptor (IFN-γR) JAK-STAT signaling pathway is required for T cells to kill cancer cells in solid tumors but not in liquid cancers.15 Meanwhile, the cell signaling reporter system containing a GAS-response element upstream of the luciferase gene (eg, Firefly luciferase; Luc2) has been widely used for detecting inter-cell IFN-γ signaling.16-18 Hence, we utilized the demonstrated IFN-γ-IFN-γR JAK-STAT GAS-Luc2 reporter system for engineering solid tumor cell lines that are capable of robustly reporting the activity of IFN-γ from activated IFN-γ-producing immune cells, including CD8+ cytotoxic T cells, CD4+ helper T cells, and CD56+ NK cells, during their interaction with solid tumor cells.
To construct these robust reporter assay systems to facilitate immune checkpoint inhibitor drug screening, we performed flow cytometry–based immune checkpoint protein profiling in over 50 tumor cell lines available at ATCC (data not shown). Based on the proteomics results, the following three cell lines, HCC827 (ATCC CRL-2868), MG-63 (ATCC CRL-1427), and NCI-H1650 (ATCC CRL-5883), were selected for their high endogenous expression of the immune checkpoint proteins, PD-L1, CD155, and B7-H3, respectively. These immune checkpoint reporter systems were designed so that the naturally high expression of the immune checkpoint molecules on the surface of the reporter cells facilitates the immune checkpoint inhibitor drug candidate binding, promoting the immune cell activation and subsequent release of IFN-γ by the immune cells, resulting in the intracellular IFN-γ-IFN-γR JAK-STAT GAS signaling activation and luciferase expression by the reporter cells (Figure 1).
Figure 1: Mechanism of action (MOA). Bioluminescence signal generated by HCC827-GAS-Luc2 cells upon T cell activation through the PD-L1 blockade. Created with BioRender.com.
Results with Materials & Methods
To establish the HCC827-GAS-Luc2 (ATCC CRL-2868-GAS-LUC2), MG-63-GAS-Luc2 (ATCC CRL-1427-GAS-LUC2), and NCI-H1650-GAS-Luc2 (ATCC CRL-5883-GAS-LUC2) cell lines, the parental cells were seeded at 1 x 105 cells/well into a 12-well culture plate and transduced with lentiviral-GAS-Luc2 plasmids in the presence of 50 μg/mL protamine sulfate (Sigma) for 24 hours. Transduction was stopped by replacing the medium with fresh culture medium. The lentiviral-GAS-Luc2 plasmids were generated by replacing the EF-1 alpha promoter with a GAS response element in a lentiviral-luciferase plasmid. The transfected cells were enriched by puromycin selection and evaluated by IFN-γ stimulation as described in the next paragraph. Following the luciferase expression assessment, single cells were isolated by automated cell sorting (Sony SH800) into 96-well plates and expanded for approximately 10-14 days until their confluency reached 70%. The growing single cell clones were then subcultured and stimulated with IFN-γ again. The clone that yielded the highest luminescence signal upon IFN-γ stimulation was chosen for the T cell–conditioned-media stimulation and co-culture experiments.
After the single-cell clonal expansion, the luminescence signal intensity upon stimulation (Figure 2) was re-evaluated by adding IFN-γ (R&D Systems) to each GAS-Luc2 modified cells. The monoclonal cells were seeded in 100 µL culture media on a 96-well plate (Corning) at the seeding density of 5.0 x 103 cells/well. Final concentrations of 0, 0.01, 0.1, 1, 10, 100, and 1,000 ng/mL IFN-γ were added to the cells followed by overnight incubation at 37°C in 5% CO2; experimental controls included media only and untreated cells. The next day, an equal volume of Bright-Glo™ Luciferase Assay Reagent (Promega) was added to the cells and the plate was incubated on a shaker for 8 minutes. The assay mixture was transferred to an opaque 96-well plate (Corning) for luminescence detection. The luminescence signals were measured by a SpectraMax® i3x (Molecular Devices). Cells were evaluated based on the average fold increase of relative luminescence (RLU) of IFN-γ-stimulated cells relative to average RLUs from untreated controls.
Initial stimulation tests of the selected monoclonal HCC827-GAS-Luc2 cell line demonstrated the highest sensitivity to IFN-γ administration, compared to the other two cell lines, exhibiting an average 15-fold increase in RLU values relative to untreated controls following 0.01 ng/mL IFN-γ administration (Figure 2A). Increased luminescence was observed with increasing IFN-γ administration, with 1,000 ng/mL IFN-γ administration resulting in a 250-fold increase in RLU values as compared to untreated controls. Similarly, the selected monoclonal MG-63-GAS-Luc2 cell line and NCI-H1650-GAS-Luc2 cell line also exhibited robust and dose-dependent luminescence signal to IFN-γ administration, demonstrating 230-fold increase and 130-fold increase, respectively, in RLUs relative to untreated controls upon 1,000 ng/mL IFN-γ administration (Figure 2B, 2C).
Figure 2: Evaluation of GAS-Luc2 cell lines via IFN-γ stimulation. Luminescence signals indicating luciferase expression by (A) HCC827-GAS-Luc2, (B) MG-63-GAS-Luc2, and (C) NCI-H1650-GAS-Luc2 cells upon JAK-STAT signaling pathway activation by IFN-γ stimulation (0.01 – 1,000 ng/mL), N=3 in all experiments. *, P < 0.05.
For T cell–conditioned-media stimulation (Figure 3), primary human CD8+ cytotoxic T cells (ATCC PCS-800-017) were cultured in RPMI1640 (ATCC 30-2001) with 10% heat-inactivated FBS (Gibco) and 50 U/mL interleukin-2 (Miltenyi Biotec). On day 0, half of the CD8+ cytotoxic T cells were activated using T Cell Activation/Expansion Kit (Miltenyi Biotec) following the manufacturer’s instructions. The remaining cells were centrifuged at 400 ×: g for 5 minutes to remove old medium and were then resuspended in fresh culture medium at a seeding density of 2.0 ×: 106 cells/mL. On day 3, the activated and non-activated T cells were harvested by centrifugation, and the supernatants were collected as activated and non-activated CD8+ cytotoxic T cell–conditioned media, respectively. Upon the preparation of the T cell–conditioned media, each GAS-Luc2 modified cell line was harvested and seeded in 100 µL culture media on a 96-well plate at the seeding density of 2.0 x 104 cells/well. The modified cells were incubated at 37°C in 5% CO2 for 1 hour to attach to the plates. After the cells settled down, an equal volume of the T cell–conditioned media was added to the cells. Cells were then incubated overnight before proceeding to the luciferase assay as described above. The activated T cell-conditioned media stimulation in HCC827-GAS-Luc2, MG-63-GAS-Luc2, and NCI-H1650-GAS-Luc2 cell lines led to 100-fold, 130-fold, and 30-fold increase in RLUs compared to untreated controls, respectively (Figure 3). In contrast, the non-activated T cell-conditioned media stimulation resulted in a 50-fold increase in RLUs in HCC827-GAS-Luc2, which previously showed the highest sensitivity to the lowest dose of IFN-γ, and more muted responses in MG-63-GAS-Luc2 and NCI-H1650-GAS-Luc2 cell lines.
Figure 3: T cell-conditioned media stimulation on GAS-Luc2 cell lines. Luminescence signals indicating luciferase expression by (A) HCC827-GAS-Luc2, (B) MG-63-GAS-Luc2, and (C) NCI-H1650-GAS-Luc2 cells upon JAK-STAT signaling pathway activation by T cell-conditioned media stimulation from non-activated and activated primary human CD8+ cytotoxic T cells. N=3 in all experiments. *, P <0.05.
Next, the ability of GAS-Luc2 cell lines to serve as reporter cell lines for immune checkpoint drug screening in solid tumors was assessed by their co-culture with primary human immune cells, including CD8+ cytotoxic T cells, CD4+ helper T cells, and CD56+ NK cells (Figure 4). The co-culture methods were optimized for each cell line: For HCC827-GAS-Luc2 cells, the GAS-Luc2 cells were added to a 96-well plate at the seeding density of 1.0 x 104 cells/well and pre-incubated for 15 minutes with five different concentrations (0, 1, 10, 100, or 1000 ng/ mL) of an anti-PD-L1 antibody, atezolizumab (Invivogen, hpdl1-mab1, MOA: PD-L1 blockade) or isotype control (Invivogen, bgal-mab1) before primary human CD8+ cytotoxic T cells (ATCC PCS-800-017) were added to the GAS-Luc2 cells at 1:1 or 1:10 target to effector cell ratio. The cells were co-cultured at 37°C in 5% CO2 for 24 or 2 hours, respectively, followed by evaluation via the luciferase assay as described above (Figure 4A & B). For MG-63-GAS-Luc2 cells, primary human CD4+ T cells (ATCC PCS-800-016) were first added to a 96-well plate at the seeding density of 1.0 x 104 cells/well and pre-incubated for 15 minutes with five different concentrations (0, 1,10, 100, or 1000 ng/ mL) of an anti-TIGIT antibody, tiragolumab (ProSci, 10-856, MOA: TIGIT blockade), or isotype control (BioLegend, 403501) before MG-63-GAS-Luc2 cells were added to the T cells at 1:1 target to effector cell ratio. The cells were co-cultured for 24 hours or 48 hours followed by evaluation via the luciferase assay (Figure 4C & D). For NCI-H1650-GAS-Luc2 cells, the GAS-Luc2 cells were seeded to a 96-well plate at the seeding density of 1.0 x 104 cells/well and pre-incubated for 15 minutes with five different concentrations (0, 1, 10, 100, or 1000 ng/ mL) of an anti-B7-H3 antibody, enoblituzumab (ProSci, 10-755, MOA: B7-H3 engaged ADCC), or isotype control (Biolegend, 403501) before primary human CD56+ NK cells (ATCC PCS-800-019) were added to the GAS-Luc2 cells at 2:1 target to effector cell ratio. The cells were co-cultured for 24 hours or 48 hours followed by evaluation via the luciferase assay (Figure 4E & F).
Figure 4: Co-culture of GAS-Luc2 cell lines with primary human immune cells. Luciferase expression from HCC827-GAS-Luc2, MG-63-GAS-Luc2, and NCI-H1650-GAS-Luc2 cells upon JAK-STAT signaling pathway activation in co-culture with primary human CD8+ cytotoxic T cells, CD4+ helper T cells, and CD56+ NK cells, respectively, in the presence of corresponding immune checkpoint antibody or isotype control (1-1,000 ng/mL). (A & B) HCC827-GAS-Luc2 and CD8+ T cells, 1:1 or 1:10 Target: Effector cell ratio, were co-cultured for 24 or 2 hours, respectively. (C & D) MG-63-GAS-Luc2 and CD4+ T cells, 1:1 Target: Effector cell ratio, were co-cultured for 24 or 48 hours. (E & F) NCI-H1650-GAS-Luc2 and NK cells, 2:1 Target: Effector cell ratio, were co-cultured for 24 or 48 hours. N=3 in all experiments. *, P < 0.05
The co-culture studies demonstrated that increasing the immune checkpoint antibody concentrations increased the luciferase expression dose-dependently in all GAS-Luc2 cell lines. In contrast, increasing the isotype control concentrations failed to induce any change in luciferase expression relative to untreated controls. HCC827-GAS-Luc2 cells showed 3- and 5-fold increase of luminescence intensity relative to untreated controls under the conditions of 24 and 2 hours of co-culture with 1:1 and 1:10 ratios of HCC827-GAS-Luc2 to CD8+ cytotoxic T cells, respectively (Figure 4A & B). MG-63-GAS-Luc2 cells exhibited 3- and 6-fold increase in luminescence intensity relative to untreated controls under the conditions of 24 and 48 hours of co-culture, respectively, with 1:1 MG-63-GAS-Luc2 to CD4+ helper T cells (Figure 4C & D). NCI-H1650-GAS-Luc2 cells displayed 2- and 3-fold increase in luminescence intensity relative to untreated controls under the conditions of 24 and 48 hours of co-culture, respectively, with 2:1 NCI-H1650-GAS-Luc2 cells to CD56+ NK cells, under the ADCC effect mediated by enoblituzumab that links NK cells to tumor cells with an increasing IFN-γ paracrine signaling from NK cells (Figure 4E & F). Overall, the data show that the GAS-Luc2 cells can effectively assess the efficacy of immune checkpoint-targeting antibodies in activating the immune cells in various co-culture conditions. Moreover, these results indicate that the application of these reporter cells is not limited to the co-culture studies with CD8+ cytotoxic T cells but can also be used in diverse co-culture studies that incorporate other types of IFN-γreleasing immune cells such as CD4+ helper T cells (Th1 & Th17) and CD56+ NK cells for various immunomodulatory drug screening.
To further demonstrate the ability of the reporter systems to mimic the in vivo CD8+ cytotoxic T cell-mediated cancer cell killing, additional co-culture conditions were evaluated using HCC827-GAS-Luc2 cells with increased CD8+ T cell populations as well as increased co-culture incubation time. Both conditions were found to greatly affect maximum luciferase expression. Increasing the ratio of CD8+ T cells to reporter cells proportionately decreased luminescence intensity after 24 hours of co-culture, with 1:5 and 1:10 HCC827-GASLuc2:CD8+ T cell ratios exhibiting no detectable luminescence signaling under 1,000 ng/ mL PD-L1 mAb administration (data not shown). Similarly, increasing the co-culturing incubation period was found to correlatedly decrease the luciferase expression when the effector cell to target cell ratio remained consistently high (1:10 HCC827-GAS-Luc2:CD8+ T cell ratio). Here, 2-, 4-, 6-, and 24-hour co-culture incubation with 1,000 ng/ mL PD-L1 mAb administration demonstrated 5-, 1-, 0.7-, and 0-fold increase in luminescence intensity relative to untreated controls, respectively (data not shown). These phenomena were attributed to the cell death of HCC827-GAS-Luc2 cells, killed by CD8+ cytotoxic T cells over time, or via elevated cytotoxicity with increased cell number of CD8+ cytotoxic T cells (data not shown). While the system was shown to effectively mimic the in vivo cancer cell killing by CD8+ cytotoxic T cells, the luminescence signal from the cancer cells naturally reached its maximum intensity before they were killed. Therefore, when designing cell-based assays using luciferase reporter systems, it is critical to consider the effect of cytotoxicity on the bioluminescence signal and optimize the assay in terms of co-culturing duration and target to effector cell ratio.
Conclusions
Based on our comprehensive proteomics data, we selected and modified three solid tumor cell lines to generate robust in vitro immune checkpoint reporting systems. HCC827, MG-63, and NCI-H1650 cell lines with high natural expression of immune checkpoint proteins, PD-L1, CD155, and B7-H3, respectively, were developed into an IFN-γ-IFN-γR JAK-STAT GAS-Luc2 reporter systems that are capable of reliably reporting the activity of IFN-γ from activated primary human immune cells that mimics the in vivo cancer cell killing events. Compared to other immune checkpoint assays on the market that use engineered cancer cell lines with ectopic overexpression of certain immune checkpoint molecules, these novel immune checkpoint assay reporting systems are much more physiologically relevant as the cancer cells that we selected have naturally high expression of relevant immune checkpoint molecules.5
To maximize assay versatility, we incorporated the IFN-γ-IFN-γR JAK-STAT GAS-Luc2 reporting system in the cancer cells so users can employ multiple kinds of human immune cells for the counterpart of the cancer cells in the full human co-culture assay. For example, we can use T cells that were newly defined by single cell sequencing (scRNAseq), CAR-T cells, B cells, and myeloid cells. Additionally, these cells could be used in combination with or without other human tumor microenvironmental cells, such as primary human fibroblasts and endothelial cells, for better modeling of the in vivo T cell-mediated cancer–killing events in the solid tumor microenvironment. All these permutations are improvements over other immune checkpoint assays on the market whose signaling reporting system is restricted to a non-cytotoxic T cell line, such as the CD4+ Jurkat T cell line with an NFAT-Luc2 reporter.6
Lastly, considering the recent studies that have demonstrated the importance of IFN-γ-IFN-γR JAK-STAT signaling for T cell–mediated killing of cancer cells specifically in solid tumors,15 our full human cancer GAS-Luc2 reporting system better supports the immune checkpoint drug discovery and development in solid tumors where the success rate of immune checkpoint blockade therapy has been markedly lower. Therefore, because of its high endogenous target marker expression, robust bioluminescent signal, and assay versatility, the ATCC GAS-Luc2 reporter cell lines are ideal for assaying and screening candidate PD-1/PD-L1, CD155/TIGIT, and B7-H3 immune checkpoint inhibitors and ADCC antibody agents.
Download a PDF of this application note
Download nowReferences
- Korman AJ, et al. The foundations of immune checkpoint blockade and the ipilimumab approval decennial. Nat Rev Drug Discov 21: 509-528, 2022. PubMed: 34937915
- Kubli SP, et al. Beyond immune checkpoint blockade: emerging immunological strategies. Nat Rev Drug Discov 20: 899-919, 2021. PubMed: 33686237
- Olson B, et al. Mouse models for cancer immunotherapy research. Cancer Discov 8: 1358-1365, 2018. PubMed: 30309862
- Neal JT, et al. Organoid Modeling of the Tumor Immune Microenvironment. Cell 175(7): 1972-1988 e16, 2018. PubMed: 30550791
- Dijkstra KK, et al. Generation of Tumor-Reactive T Cells by Co-culture of Peripheral Blood Lymphocytes and Tumor Organoids. Cell 174(6): 1586-1598 e12, 2018. PubMed: 30100188
- Versteven M, et al. A versatile T cell-based assay to assess therapeutic antigen-specific PD-1-targeted approaches. Oncotarget 9(45): 27797-27808, 2018. PubMed: 29963238
- Ribas A, Wolchok JD. Cancer immunotherapy using checkpoint blockade. Science 359(6382): 1350-1355, 2018. PubMed: 29567705
- Sharma P, Allison JP. The future of immune checkpoint therapy. Science 348(6230): 56-61, 2015. PubMed: 25838373
- Yang S, Wei W, Zhao Q. B7-H3, a checkpoint molecule, as a target for cancer immunotherapy. Int J Biol Sci 16(11): 1767-1773, 2020. PubMed: 32398947
- Zhou W-T, Jin W-L. B7-H3/CD276: An Emerging Cancer Immunotherapy. Front Immunol 12: 701006, 2021. PubMed: 34349762
- Kučan Brlić P, et al. Targeting PVR (CD155) and its receptors in anti-tumor therapy. Cell Mol Immunol 16(1): 40-52, 2019. PubMed: 30275538
- Braun M, et al. CD155 on Tumor Cells Drives Resistance to Immunotherapy by Inducing the Degradation of the Activating Receptor CD226 in CD8(+) T Cells. Immunity 53(4): 805-823.e15, 2020. PubMed: 33053330
- Chen DS, Mellman I. Elements of cancer immunity and the cancer-immune set point. Nature 541(7637): 321-330, 2017. PubMed: 28102259
- Dall’Olio FG, et al. Tumour burden and efficacy of immune-checkpoint inhibitors. Nat Rev Clin Oncol 19(2): 75-90, 2022. PubMed: 34642484
- Larson RC, et al. CAR T cell killing requires the IFNγR pathway in solid but not liquid tumours. Nature 604(7906): 563-570, 2022. PubMed: 35418687
- Platanias LC. Mechanisms of type-I- and type-II-interferon-mediated signalling. Nat Rev Immunol 5(5): 375-86, 2005. PubMed: 15864272
- Brasier AR, Tate JE, Habener JF. Optimized use of the firefly luciferase assay as a reporter gene in mammalian cell lines. Biotechniques 7(10): 1116-22, 1989. PubMed: 2698191
- Grewal T, et al. Identification of a gamma-interferon-responsive element in the promoter of the human macrophage scavenger receptor A gene. Arterioscler Thromb Vasc Biol 21(5): 825-31, 2001. PubMed: 11348881