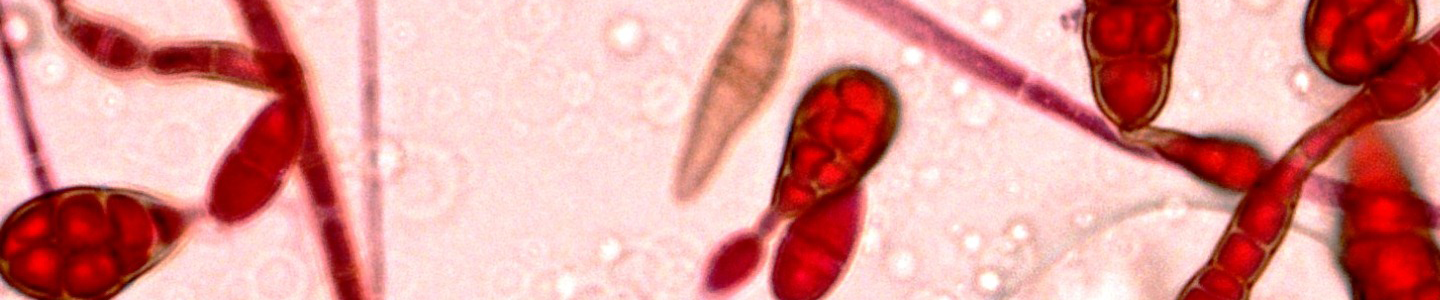
This guide contains general technical information for mycological growth, propagation, preservation, and application. Additional information on yeast and fungi can be found in Introductory Mycology by Alexopoulos et al.1
Table of Contents
Getting Started with an ATCC Mycology Strain
Mycological Growth and Propagation
Growth Media
Preservation
Biosafety and Disposal
Mycological Authentication
Mycological Applications
Glossary
References
Download a PDF of our mycology guide
Download nowGetting Started with an ATCC Mycology Strain
ATCC mycology strains are shipped either frozen on dry ice, in plastic or glass cryopreservation vials, as lyophilized cultures in glass ampoules or serum vials, or as live cultures in test tubes. Upon receipt of frozen cells, immediately revive cells by thawing and subsequently transferring cells to an appropriate growth medium. If this is not possible, store frozen vials in liquid nitrogen vapor (below -130°C). Alternatively, the frozen material can be stored between -70°C and -80°C for short periods (1 to 5 days); however, viability will decline at temperatures above -130°C. Freeze-dried cultures can be stored safely at 4°C or colder. Upon receipt, rehydrate or dilute freeze-dried cultures using sterile distilled water or the appropriate medium and incubate under the conditions specified on the product sheet. Live cultures should be immediately transferred to tubes or plates containing the recommended medium and incubated under the appropriate growth conditions. Under no circumstances should live cultures be stored at refrigerated or standard freezer temperatures as this may result in the death of the culture.
Product Sheet
A product sheet that contains detailed information on the processing and expansion of materials, as well as ideal growth and propagation conditions, are provided for each ATCC mycology strain. This product sheet, as well as additional information, can be found on the ATCC website or can be requested from the ATCC Product Experience team.
Preparation of Medium
In advance, prepare the appropriate medium and additional supplements necessary for yeast and fungal revival and growth. Additionally, ensure that your incubator is set to maintain the optimal growth conditions of the strain. Information for the formulation and preparation of the media and incubation conditions for these products is available on the ATCC website.
Opening Glass Ampoules
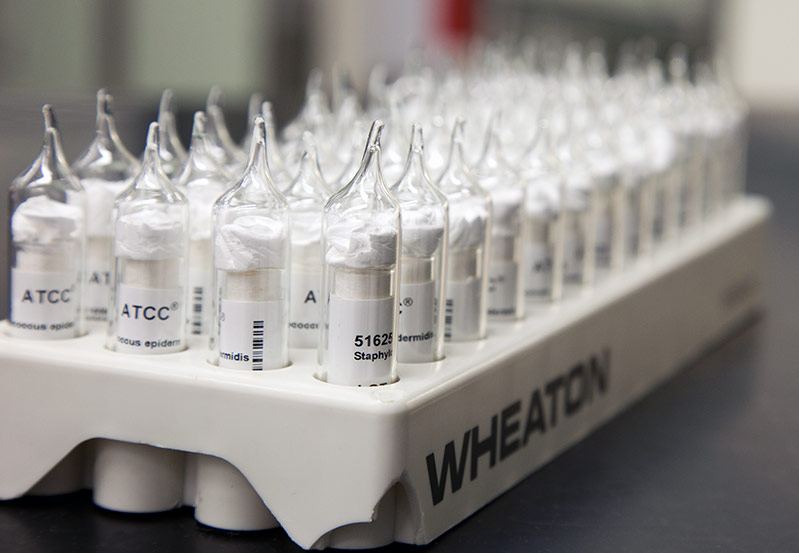
Overview
All cultures should be considered potentially hazardous and should be opened by individuals trained in microbiological techniques working in facilities with containment requirements appropriate for the biosafety level of the cultures. ATCC recommends that the handling or opening of glass ampoules be performed in a biological safety cabinet. If this is not possible, wear protective clothing, gloves, a face shield or safety goggles, and hold the vial away from your body. Ensure that all empty vials are sterilized before disposal.
- Opening Double-Vial Preparations
- Heat the tip of the vial in a flame.
- Add a few drops of water on the hot tip to crack the glass.
- Strike the end of the vial with a file or pencil to remove the tip.
- Remove the insulation and inner vial with sterile forceps. Gently raise the cotton plug.
- Opening Single-Vial Preparations
- To recover the cell suspension from the glass ampoule, score the neck of the ampoule with a small, sterile file.
- Disinfect the outside of the ampoule with freshly prepared 70% ethanol or dip it into a beaker of freshly prepared 70% ethanol.
- Wrap the ampoule within several folds of a sterile towel or gauze to dry residual ethanol.
- Working in a laminar flow hood, hold the vial upright and snap open the vial. Ensure that your gauze does not become too wet with ethanol, or alcohol could be sucked into the culture when the vacuum is broken. Rehydrate the material immediately.
Initiating Frozen Cultures
- Prepare a sterile test tube that contains the recommended medium for growth as listed in the product sheet. Ensure that the medium contains all necessary supplements or components and is equilibrated for temperature and pH.
- Thaw the sample vial via gentle agitation in a water bath that is set to 25°C to 30°C. Thawing will be rapid; approximately 5 minutes or until all ice crystals have melted.
- Remove the vial from the water bath and decontaminate the outer surface using freshly prepared 70% ethanol. Follow strict aseptic conditions in a laminar flow hood for all further manipulations.
- Unscrew the top of the vial and transfer the entire contents to a sterile test tube or plate containing the appropriate growth medium. For yeast cells in suspension, cultures can be spread-plated on the medium to promote the growth of individual colonies. Note that many frozen ATCC mycology items contain agar plugs made from fully grown culture.
- Incubate cultures under the appropriate temperature and atmospheric conditions as recommended on the product sheet.
- Examine cultures after the recommended incubation period. The incubation period will vary between strains and is listed on the product sheet (See: NOTE 1).
NOTE 1:
Following recovery from cryopreservation or lyophilization, some mycological strains may exhibit a prolonged lag phase. These strains will require an extended incubation period.
Initiating Lyophilized Cultures
- Using a Pasteur pipette, aseptically add 0.5 to 0.9 mL of sterile water to the freeze-dried material. Mix well.
- Transfer the entire suspension to a test tube containing 6 mL of sterile water.
- Allow the culture to soak for 50 to 60 minutes before transferring to solid media. For some strains, prolonged rehydration is recommended.
- Incubate cultures under the appropriate temperature and atmospheric conditions as recommended on the product sheet. Additional agar medium can be inoculated by transferring 0.5 mL of the primary culture to additional secondary cultures.
- Most freeze-dried cultures will grow within a few days (See: NOTE 1).
Initiating Test Tube Cultures
- Upon receipt of the live culture, aseptically transfer the culture from the provided test tube into a test tube or plate containing the recommended medium.
- Incubate the test tube or plate under the recommended atmospheric conditions and temperature.
- Examine cultures after the recommended incubation period. The incubation period will vary between strains and is listed on the product sheet.
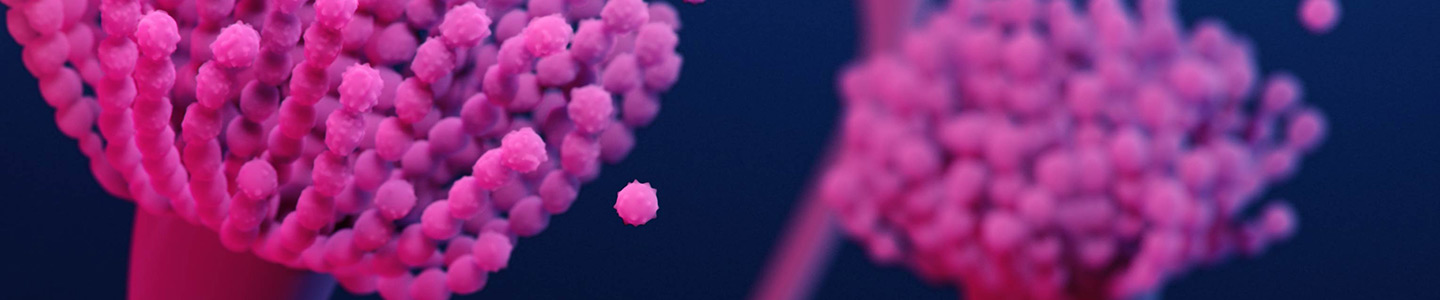
Mycological Growth and Propagation
Properties of Mycology Phyla
Currently, there are an estimated 1.5 million species in the fungal kingdom, including various yeasts, rusts, smuts, mildews, molds, mushrooms, and toadstools.2 These eukaryotic organisms are abundant worldwide and encompass a large diversity of taxa that vary in morphology, reproduction, and ecology. Below, we discuss some of the distinguishing characteristics of known fungal phyla (Table 1).
Table 1. Fungal Phyla
Phylum | Common Names | Distinguishing characteristics |
---|---|---|
Chytridiomycota | Chytrids | Zoospores with a single, posterior flagellum. Life cycle with zygotic meiosis. |
Glomeromycota | Arbuscular mycorrhizal fungi, Glomales, Glomerales | Formation of arbuscules |
Zygomycota | Sugar Mold, Pin Mold | Produces thick-walled resting spores (zygospores) |
Blastocladiomycota | Blastocladialeans | Production of zoospores. Life cycle with sporic meiosis. |
Ascomycota | Ascomycetes, Sac Fungi | Formation of an ascus |
Basidiomycota | Basidiomycetes, Club Fungi | Production of sexual spores, termed basidiospores |
Neocallimastigomycota | N/A | Production of zoospores. Presence of hydrogenosome instead of mitochondria. |
Microsporidia | N/A | Unicellular parasite. Presence of mitosome instead of mitochondria. |
Oomycota | Oomycetes, Water Mold | Production of oversized oogonia |
Chytridiomycota
The phylum Chytridiomycota contains a number of genera including Spizellomyces, Chytriomyces, and Rhizophydium (Table 2). These organisms, known as chytrids, are the smallest and simplest fungi with unicellular or mycelial thalli composed of chitinous cells.3 They are distinguished by having zoospores with a single, posterior flagellum.1
Like many fungal species, chytrids predominantly demonstrate saprotrophic nutrition with the ability to degrade chitin and keratin. However, several species have been found to be pathogenic on plants, animals, and other fungal species.4 Strains within this phylum commonly inhabit freshwater ecosystems, which aids in the motile stages of both the asexual and sexual phases of the life cycle. The chytrid life cycle has the ability to alternate between haploid and diploid stages between generations, with sexual reproduction as haploid-dominant and asexual reproduction as diploid-dominant. During sexual reproduction, the haploid thallus forms male and female gametangia from which motile, flagellated gametes are released and subsequently fuse to form a diploid zygote. Upon germination, the zygote will produce a diploid thallus with two types of sporangia; a zoosporangia that releases diploid zoospores to reproduce asexually, and a sporangia generated by zygotic meiosis that releases haploid zoospores to form sexually reproducing haploid thalli.1
Table 2. Chytridiomycota Examples
ATCC Number | Organism | Designation | Application |
---|---|---|---|
60989 | Allochytridium luteum | Barr 463 | Type strain |
28165 | Chytriomyces hyalinus | SOA 1 | Recommended for classroom demonstration |
90967 | Entophlyctis luteolus | L-129 | Type strain |
MYA-2894 | Monoblepharella sp. | M# 15 [JEL 485] | Sequenced mitochondrial genome |
MYA-4069 | Rhizophydium globosum | JEL 222 | Type strain |
18786 | Rhizophydium sphaerotheca | 70 [IMI 143633] | Recommended for classroom demonstration |
MYA-1381 | Aurantiochytrium limacinum | SR21 [IFO 32693] | Type strain |
18671 | Spizellomyces plurigibbosus | 33 [CBS 668.73, IMI 143638] | Type strain |
48900 | Spizellomyces punctatus | 117 [NG-3] | Type strain, genome sequencing strain |
18785 | Triparticalcar arcticum | 59 [CBS 666.73, IMI 143636] | Type strain |
Glomeromycota
Members of the phylum Glomeromycota are biotrophs found worldwide in terrestrial ecosystems, where they form a unique symbiotic relationship with the roots of local plant species. In this symbiosis, Glomeromycota species form arbuscular mycorrhizas with the roots or thalli of land plants, to obtain carbon and energy. However, there is some evidence that Glomeromycota may exist independently, without forming a symbiotic relathioship.5
Fungi within this phylum form coenocytic mycelia that reproduce asexually through the formation of spores. Under favorable conditions, the spores germinate, form arbuscules with plant roots, and establish new mycorrhizal symbiotic relationship.6 Examples of Glomeromycota genera include Geosiphon, Glomus, and Acaulospora.
Zygomycota
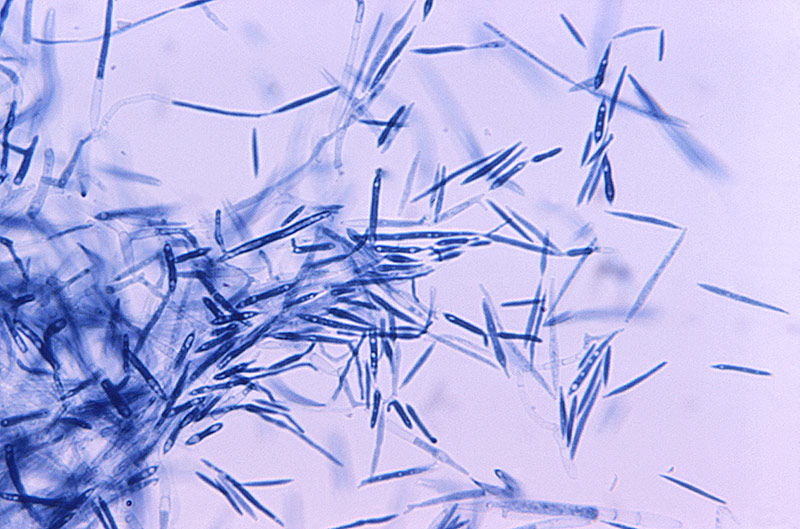
Image of Ustilago maydi courtesy of Dr. Lucille Georg, CDC
The phylum Zygomycota contains many genera including Conidiobolus, Erynia, and Mucor (Table 3). In recent years, fungi traditionally classified into Zygomycota were reorganized taxonomically.13 Species within the Zygomycota include destructive sugar molds or pin molds, though many species within this phlylum can form symbiotic relationships with plants. The Zygomycota normally grow as mycelia or as filaments, each composed of chitin-chitosan walled cells.1
Members of this terrestrial phylum are able to reproduce both sexually and asexually, with sexual reproduction as haploid-dominant and asexual reproduction as diploid-dominant. During asexual reproduction, asexual spores, called sporangiospores, are produced in sporangia. Following germination, sporangiospores form somatic hyphal structures. During sexual reproduction, different mating strains (progametangia) grow toward each other and fuse to form a zygote. The zygote will then develop to form a zygospore, which is a thick-walled resting spore characteristic of the phylum. The zygospore will remain dormant for a period of time before beginning the reproductive cycle again.1
Table 3. Zygomycota Examples
ATCC Number | Organism | Designation | Application |
---|---|---|---|
24418 | Conidiobolus obscurus | [CBS 182.60] | Type strain |
32803 | Entomophthora destruens | NRRL 3727 [CBS 208.65] | Type strain |
34698 | Erynia blunckii | Nr. 2 [CBS 194.77, IMI 211910] | Type strain |
208867 | Eryniopsis ptychopterae | ARSEF 2671 [KVL48] | N/A |
MYA-4580 | Furculomyces boomerangus | AUS-42-7 | Homothallically reproducing Harpellales |
36965 | Mortierella alpina | 84 | Degrades paper-mill wastes |
MYA-4767 | Mucor ellipsoideus | UTHSC 02-2090 [FMR 10021, CBS 126271] | Type strain |
MYA-4621 | Rhizopus oryzae | FGSC 9543 [RA99-880] | Genome sequencing strain (Broad Institute, USA) |
MYA-4582 | Smittium angustum | AUS-126-30 | Trichomycete gut fungus |
208865 | Zoophthora radicans | ARSEF 4784 [KVL610] | N/A |
Blastocladiomycota
The phylum Blastocladiomycota is currently recognized as a “sister phylum” to both the Chytridiomycota and Neocallimastigomycota.10 Originally, the blastocladialean fungi were classified under the phylum Chytridiomycota, but upon recent DNA analysis, they have been designated as a distinct phylum.10 The Blastocladiomycota are zoosporic fungi that inhabit freshwater or soil. Similar to the Chytridiomycota, the Blastocladiomycota species are either saprotrophic or parasitic on algae, land plants, fungi, and invertebrates.
The Blastocladiomycota differ from their Chytridiomycota sister phylum in their life cycle. Unlike the Chydriomycota, which utilize zygotic meiosis, the Blastocladiomycota use sporic meiosis, indicating that the zygote divides mitotically to produce a multicellular diploid sporophyte.11 This sporophyte creates spores by meiosis, which can then divide mitotically to produce haploid individuals called gametophytes. Current examples of Blastocladiomycota genera include Allomyces, Blastocladia, and Coelomomyces (Table 4).
Table 4. Blastocladiomycota Examples
ATCC Number | Organism | Designation | Application |
---|---|---|---|
10984 | Allomyces javanicus | [CBS 600.78] | N/A |
Allomyces reticulatus | [Cal 70, UC 1445835] | N/A | |
44886 | Allomyces macrogynus | asn 1 | Auxotrophic mutant of ATCC 38327 |
Ascomycota
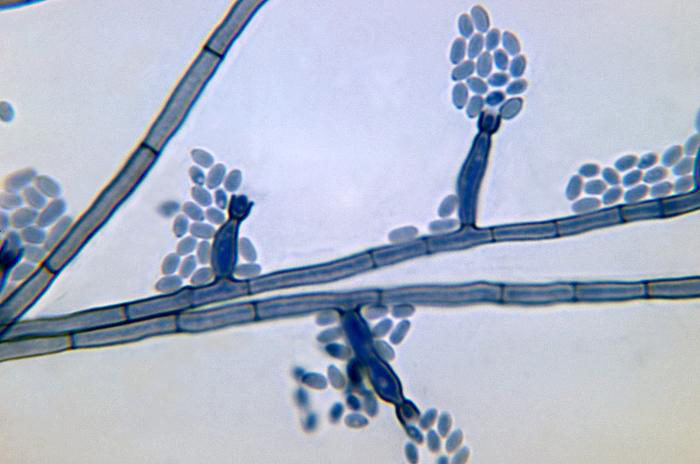
Image of Phialophora verrucosa courtesy of Dr. Libero Ajello, CDC
Members of the phylum Ascomycota are commonly known as the sac fungi due to their defining feature, the ascus, which contains nonmotile ascospores developed by free-cell formation and resembles a sac.1 This structure is used in the sexual reproduction stage of the Ascomycota life cycle. Following germination of the ascospore, a somatic hypha is formed, which subsequently develops into a condiophore. The condiophore structure is part of the asexual, dominant stage of the Ascomycota life cycle. The condiophore is responsible for the production and rapid spread of vegetative reproductive spores known as conidia.1
Many species within this phylum are known to grow as filamentous mycelium, and are made up of cells containing chitin and β-glucans in the cell wall. However, a number of Ascomycota species, such as those within the genera Candida and Saccharomyces, commonly grow as yeasts (Table 5). These latter organisms reproduce by budding. Members of the Ascomycota are of particular relevance to humans as they are sources of antibiotics and are used in the production of food products such as bread, cheese, beer, and wine. However, other members of this phylum are known pathogens to a variety of species. Plant pathogens within this phylum cause diseases such as apple scab, rice blast, and Dutch elm disease.1, 7
Table 5. Ascomycota Examples
ATCC Number | Organism | Designation | Application |
---|---|---|---|
10231 | Candida albicans | 3147 [CBS 6431, CCY 29-3-106, CIP 48.72, DSM 1386, IFO 1594, NCPF 3179, NCYC 1363, NIH 3147, VTT C-85161] | Reference strain for many applications |
6205 | Chaetomium globosum | QM 459 [1042.4, CBS 148.51, CBS 161.52, CEB 1218.1, CEB 1218.2, CECT 2701, DSM 1962, IFO 6347, IMI 45550, MUCL 1984, NRRL 1870, UPSC 3159, VTT D-81079] | Reference strain for various testing |
76902 | Dipodascopsis tothii | CBS 759.85 [CSIR 31, NRRL Y-12690] | Produces prostaglandin during ascosporogenesis |
26050 | Gerlachia nivalis | N/A | Snow mold, pathogenic to rye or grass roots |
MYA-4619 | Neurospora crassa | FGSC 9013 [OR74A, N10} | Genome strain and genetic studies |
26475 | Neurospora crassa | 74A-T28-M1 | Genetic studies |
204508 | Saccharomyces cerevisiae | S288C | Genome sequencing strain and parent strain of many genetic strains |
24843 | Schizosaccharomyces pombe | HFBDG41 | Genome strain and genetic model strain |
13631 | Trichoderma reesei | QM 6a [CBS 383.78, IMI 192654, IMI 45548, T.V. B117] | Enzyme production, reference strain, genome strain |
18377 | Xanthoria parietina | N/A | Lichen symbiont |
Basidiomycota
The phylum Basidiomycota is a diverse group of fungi, known as club fungi, that contains organisms such as mushrooms, boletes, puffballs, earthstars, stinkhorns, bird’s-nest fungi, jelly fungi, bracket or shelf fungi, rusts, and smuts.1 Examples of genera include Agaricus, Pleurotus, and Ganoderma (Table 6). These filamentous fungi are primarily characterized by the production of sexual spores, termed basidiospores, localized on the outside of specialized spore-producing structures called a basidium. In most basidiomycetes, with the exception of rusts and smuts, the basidium is produced in a fruiting body known as a basidiocarp. Sexual production of Basidiomycota culminates in the production of basidiospores from the basidiocarp. In contrast, asexual reproduction in the basidiomycetes takes place by way of budding through the fragmentation of mycelium and the production of conidia, arthrospores, or oidia. This latter form of reproduction is the sole method used by some basidiomycetes, including many yeasts.1
The basidiomycetes are found in most terrestrial ecosystems, as well as freshwater and marine habitats.8 These fungi help degrade dead organic matter and thus have a significant role in maintaining their ecosystem. The basidiomycetes are also important in human affairs as some species function as pathogens, or are inherently toxic, whereas other species can be used as a source of food.1 Smuts, for example, are cereal and crop pathogens that have been found to devastate grass crops such as barley, wheat, oats, and maize.9 In contrast, many mushroom species, such as shiitake and oyster mushrooms, are routinely consumed.1
Table 6. Basidiomycota Examples
ATCC Number | Organism | Designation | Application |
---|---|---|---|
36981 | Agaricus bisporus | D621 | Commercial white mushroom |
34620 | Boletus edulis | CBS 455.69 | Edible mushroom |
MYA-4618 | Coprinopsis cinerea | FGSC 9003 [okayama7#130] | Mushroom, genome sequencing |
200597 | Ganoderma licidum | N/A | N/A |
MYA-4612 | Malassezia globosa | CBS 7966 [ATCC 96807, GM 35] | Genome strain, opportunistic pathogen to humans |
MYA-4611 | Malassezia restricta | CBS 7877 [ATCC 96810, JCM 14890, RA 42.2C, NBRC 103918] | Genome strain, opportunistic pathogen to humans |
MYA-4764 | Phanerochaete chrysosporium | 2-2786 | Hite rot fungus, genome sequencing strain |
96999 | Pleurotus ostreatus | CCB 004 | Grey oyster mushroom |
MYA-4550 | Sporobolomyces salmonicolor | VITEK 301525 [MUCL 51628] | Reference strain for VITEK2 |
20509 | Cutaneotrichosporon oleaginosus | D | Oil production from cellulosic degradation |
Neocallimastigomycota
The phylum Neocallimastiglomycota is currently recognized as a “sister phylum” to both the Blastocladiomycota and Chytridiomycota. This phylum is composed of anaerobic fungi found in the rumen and hindgut of many larger mammalian herbivores.10 Species within the Neocallimastiglomycota phylum play an essential role in the digestion of fiber in their mammalian host, producing a polysaccharide-degrading enzyme used to breakdown plant materials.12
Much like their sister phyla, the Neocallimastiogomycota produce flagellated zoospores. These zoospores, however, differ in that they lack the nonflagellated centriole found in most chytrids.13 In addition to this difference, the Neocallimastigomycota also lack mitochondria. Rather, they possess an organelle called a hydrogenosome that produces molecular hydrogen as a byproduct of energy generation under anaerobic conditions.14 Examples of Neocallimastigomycota include Orpinomyces, Piromyces, Neocallimastix, Caecomyces, and Anaeromyces.
Microsporidia
The phylum Microsporidia is composed of spore-forming unicellular parasites. Several examples of this phylum include the genera Enterocytozoon, Pleistophora, and Encephalitozoon. These fungal organisms, once believed to be protists, primarily infect arthropods and fish. However, Microsporidia also have medical relevance due to their ability to infect immunocompromised individuals. These organisms are unique in that they have some of the smallest genomes among eukaryotic organisms. In addition, Microsporidia species lack mitochondria; rather, they possess mitosomes.
Microsporidia replicate within the intestinal system of their host. Consumed microsporidial spores germinate and swell to the point of rupturing, which forces an internal filament to rapidly eject infectious content, termed sporoplasm, into surrounding host cells. Once inside the host, sporoplasm grows to form the Microsporidia organism; upon maturation, the organism produces new spores, which are spread to new hosts via fecal matter contamination and inhalation. This life cycle can vary considerably between species, varying in both asexual and sexual reproduction.15
Oomycota
Species within the phylum Oomycota, commonly known as water molds, are recognized as pseudo- fungal, pathogenic, eukaryotic microorganisms. Examples of Oomycota include species within the genera Phytophthora, Pythium, and Saprolegnia (Table 7). Generally, these organisms are found worldwide, resulting in devastating plant diseases such as the potato blight and sudden oak death.1 Oomycetes appear either unicellular or filamentous and branching, with the latter morphology rarely containing septa.16 The cell wall structure of oomycetes consists of cellulose and β-1,3- and β-1,6-glucans.1
The oomycetes reproduce both sexually and asexually. Sexual reproduction in oomycetes is usually heterogametangic; in most species, the gametangia differentiate into small hyphal-like male structures, termed antheridia, and larger globose female structures, termed oogonia.1 These sexual structures develop either from different thalli or from the same thallus; although, gametangia produced on the same thallus may not be compatible. During sexual reproduction, developing antheridia are attracted to oogonia by hormones, giving rise to the production of fertilization tubes. A haploid nucleus resulting from meiosis within the antheridium is introduced into the oosphere using the fertilization tube, fusing with the oosphere nucleus. The fertilized oosphere subsequently develops into an oospore within the oogonium where it will eventually be released following maturation. Upon germination, the oospore will develop into a diploid thallus.1, 17
During asexual reproduction, most oomycetes reproduce using heterokont zoospores. Depending on the species of oomycete, these spores will either develop into sporangia or a vesicle that protrudes from the sporangium. In many species, two morphologically distinct forms of zoospores are produced: the primary zoospore and the secondary zoospore. The primary zoospore is pyriform in shape, with a flagella attached at the anterior end of the spore. The secondary zoospore, produced by most oomycetes, is reniform in shape, with a flagella laterally inserted in an indentation on the spore surface.1
Table 7. Oomycota Examples
ATCC Number | Organism | Designation | Application |
---|---|---|---|
MYA-4127 | Phytophthora infestans | T30-4 | Genome sequencing strain |
MYA-4040 | Phytophthora megakarya | p42 [22H7, 203532] | Pathogenic to cacao trees |
MYA-2949 | Phytophthora ramorum | Pr-102 | Genome sequencing strain |
MYA-4756 | Phytophthora sojae | p236 [P6497, 28F9] | Genome sequencing strain |
66260 | Pythium carolinianum | 85-54 | Pathogenic to cherry seeds |
28251 | Pythium insidiosum | K169-B91 [A-25710] | Pathogenic to horses |
201957 | Pythium oligandrum | 91-4 | Potential biological control against root pathogens |
36145 | Saprolegnia diclina | P203 | Parasitic on Perca fluviatilis |
MYA-399_TT | Saprolegnia salmonis | NJM 9851 | Pathogenic to salmon |
38488 | Saprolegnia shikotsuensis | SANK 23 177 | Fish parasite |
Mycological Growth Conditions
Nutrition
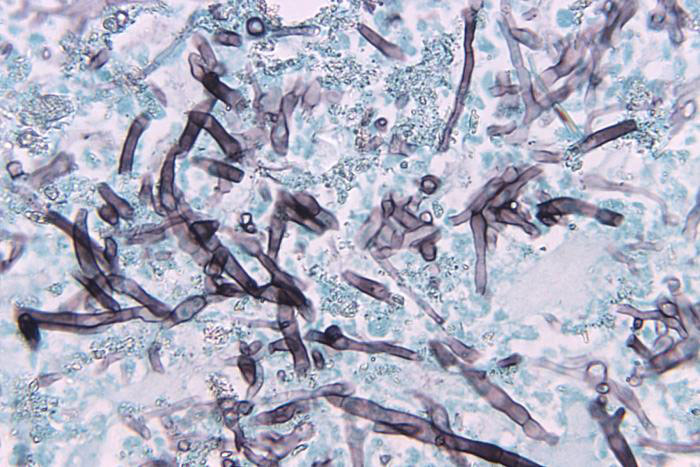
Image of Bipolaris hawaiiensis courtesy of Dr. Libero Ajello, CDC
The fungal kingdom is predominantly composed of heterotrophic saprobes that survive by absorbing dead organic matter as a source of nutrition. To consume organic matter, fungi must release digestive enzymes to break down large carbohydrates, proteins, and lipids into smaller usable molecules that can easily be absorbed across both the cell wall and plasma membrane. The ability for fungus to survive in their environment depends heavily on the type of digestive enzymes produced and released. Ultimately, for this process to be possible, the fungal species must grow in a location that has a source of water to serve as a medium for diffusion.1
A considerable number of fungal strains survive as parasites on plants, animals, or other fungi. Many of these species are still capable of a saprotrophic life style, whereas others are obligate parasites. Fungal species that survive as obligate parasites are fairly restricted in their nutritional needs, often requiring the living protoplasm from a specific species for survival.1
Environmental Conditions
Fungal growth depends heavily on the moisture, temperature, pH, and atmospheric conditions of the environment. As described below, these requirements can vary significantly between species.1
- Moisture
All fungal species require the presence of moisture; however, the required amount and osmotic property of water varies between species. Some species, for example, require only a minimal amount of water to aid in absorption, whereas other species are able to survive on substrates that have high salt content. - Temperature
Most fungal species can survive at temperatures ranging from 10°C to 40°C, with optimal temperatures between 25°C and 30°C. Other extremophilic species are able to survive outside these temperature ranges under either thermophilic or psycrophilic conditions. Ideal growth temperature will vary between species (See: NOTE 2). - pH
The requirements for environmental pH can vary significantly between species. Many fungal species thrive at lower pH levels of 4-7. This pH range, however, can be affected by the generation of byproducts during metabolism. If the pH level falls outside of the optimal range, fungal growth can be affected. - Atmosphere
Typically, most fungal species are aerobic, requiring the presence of oxygen for survival. However, the level of required oxygen can vary between species, ranging from obligate anaerobic conditions, as seen with the Neocallimastiglomycota; facultative anaerobic conditions, required by some species such as Trichocladium canadense; and aerobic conditions, as seen with most fungal organisms.
NOTE 2:
Regularly calibrate the temperature control system of incubators. Use an alarm system when possible to warn against temperature fluctuations above or below the optimum setting.
Mycological Propagation
Propagation of mycology strains can vary significantly between species. Below, we describe the general procedure for the propagation of yeasts and fungi. Information regarding the recommended medium and growth conditions can be found on the product sheet. The product sheet, as well as additional information, can be found on the ATCC website or can be requested from the ATCC Product Experience team. Additional information can be found in the fourth edition of Introductory Mycology.1
Propagation of Frozen Cultures
Frozen ampoules packed in dry ice should either be thawed immediately or stored in liquid nitrogen. If liquid nitrogen storage facilities are not available, frozen ampoules may be stored at or below -70°C for approximately one week. Do not under any circumstance store frozen ampoules at refrigerator freezer temperatures (generally -20°C). Storage of frozen material at this temperature will result in the death of the culture.
- To thaw a frozen ampoule, place in a 25°C to 30°C water bath and gently agitate, until just thawed (approximately 5 minutes). Immerse the ampoule just deep enough to sufficiently cover the frozen material.
- Immediately after thawing, wipe down ampoule with freshly prepared 70% ethanol and aseptically transfer at least 50 µL (or 2-3 agar cubes) of the content onto a plate or broth with the recommended medium.
- Incubate the inoculum/strain at the recommended temperature and environmental conditions.
- Inspect for growth of the inoculum/strain regularly. Signs of viability can be determined through visual inspection after the appropriate incubation period as listed on the product sheet. However, the time necessary for significant growth will vary from strain to strain.
Propagation of Lyophilized Cultures
- Open an ampoule according to the product sheet.
- From a single test tube of sterile distilled water (5 to 6 mL), withdraw approximately 0.5 to 1.0 mL with a sterile pipette and apply directly to the pellet. Stir to form a suspension.
- Aseptically transfer the suspension back into the test tube of sterile distilled water.
- Let the test tube sit at room temperature (25°C) undisturbed for at least 2 hours; longer (eg, overnight) rehydration might increase viability of some fungi.
- Mix the suspension well. Use several drops (or make dilutions if desired) to inoculate recommended solid or liquid medium. Include a control that receives no inoculum.
- Incubate the inoculum at the recommended propagation conditions.
- Inspect for growth of the inoculum/strain regularly. Viability can be determined through visual inspection after the appropriate incubation period as listed on the product sheet. However, the time necessary for significant growth will vary from strain to strain.
Propagation of Live Cultures
Do not under any circumstance store live test tube cultures at freezer temperatures (generally -20°C). Storage of material at this temperature may result in the death of the culture.
- Using sterile technique, transfer a piece of the fungal tissue to tubes or plates of the recommended medium. New cultures may grow more quickly if mycelium is placed in contact with the fresh medium.
- Incubate cultures under the recommended growth conditions.
- Inspect for growth of the inoculum/strain regularly. Viability can be determined through visual inspection after the appropriate incubation period as listed on the product sheet. However, the time necessary for significant growth will vary from strain to strain.
Propagation of Specific Mycology Species
Candida albicans (Phylum Ascomycota)
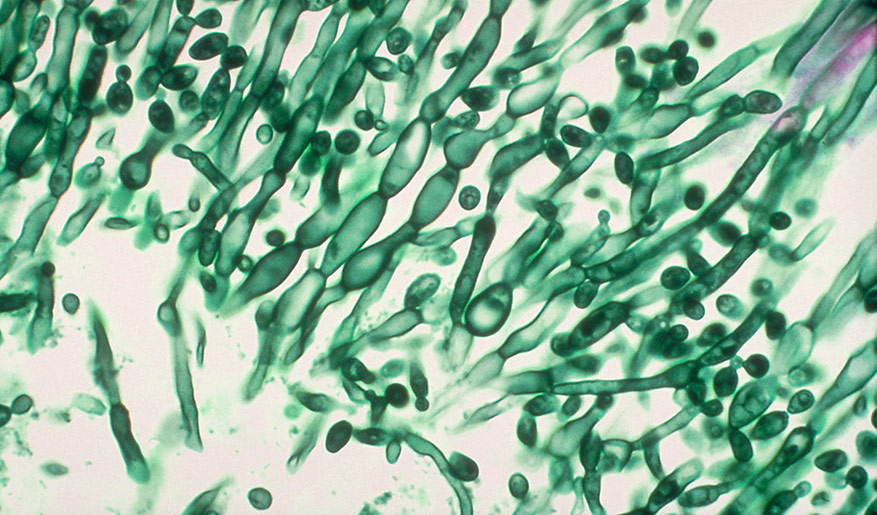
Image of Candida albicans courtesy of CDC
Candida albicans cultures are preserved either through lyophilization or cryopreservation. Depending on the method the culture was preserved, the propagation procedure may vary. Once the culture has been expanded, C. albicans can be maintained on agar and kept at 4°C for about a week. For long-term storage, cultures should be preserved for future use. To propagate lyophilized cultures, ATCC recommends the following procedure:
- Open an ampoule according to the product sheet.
- From a single test tube of sterile distilled water (5 to 6 mL), withdraw approximately 0.5 to 1.0 mL with a sterile pipette and apply directly to the pellet. Stir to form a suspension.
- Aseptically transfer the suspension back into the test tube of sterile distilled water.
- Let the test tube sit at room temperature (25°C) undisturbed for 1-2 hours.
- Mix the suspension well. Use several drops (or make dilutions if desired) to inoculate the recommended solid or liquid medium. Include a control that receives no inoculum.
- Incubate the inoculum at the recommended propagation conditions. For most C. albicans strains, an aerobic atmosphere at 20-25°C is usually recommended.
- Inspect for growth of the inoculum/strain regularly. Viability is noticeable typically after 1-2 days of incubation. However, the time necessary for significant growth will vary from strain to strain.
Saccharomyces cerevisiae (Phylum Ascomycota)
Saccharomyces cerevisiae is a species of yeast used extensively as a eukaryotic model organism in molecular and cell biology. S. cerevisiae cultures are preserved either through lyophilization or cryopreservation. Depending on the method the culture was preserved, the propagation procedure may vary. Once the culture has been expanded, S. cerevisiae can be maintained on agar and kept at 4°C for about a week. For long-term storage, cultures should be preserved for future use. To propagate frozen cultures, ATCC recommends the following procedure:
- To thaw a frozen ampoule, place in a 25°C to 30°C water bath, until just thawed (approximately 5 minutes). Immerse the ampoule just deep enough to sufficiently cover the frozen material. Do not shake the ampoule.
- Immediately after thawing, wipe down ampoule with freshly prepared 70% ethanol and aseptically transfer at least 50 µL of the content onto a plate or broth with the recommended medium.
- Incubate the inoculum/strain at the recommended temperature and environmental conditions. S. cerevisiae is usually propagated under aerobic conditions at 20-25°C.
- Inspect for growth of the inoculum/strain regularly. The sign of viability is typically noticeable after 1-2 days of incubation. However, the time necessary for significant growth will vary from strain to strain.
Aspergillus brasiliensis (Phylum Ascomycota)
Image of Aspergillus sp. courtesy of Janice Haney Carr, CDC
During the propagation of A. brasiliensis, sporulation may be inhibited in plates that are sealed completely with tape or film. To enhance sporulation, propagate the strain in an aerobic atmosphere on growth media with limited nutrients. Colonies grown directly from rehydrated spores may exhibit sectoring with areas of varying levels of sporulation. To reduce sectoring, ATCC recommends using freshly produced spores to seed plates. ATCC recommends the following procedure below for the propagation of A. brasiliensis:
- Open an ampoule according to the product sheet.
- From a single test tube of sterile distilled water (5 to 6 mL), withdraw approximately 0.5 to 1.0 mL with a sterile pipette and apply directly to the pellet. Stir to form a suspension.
- Aseptically transfer the suspension back into the test tube of sterile distilled water.
- Let the test tube sit at room temperature (25°C) undisturbed for at least 2 hours. Please note that longer (eg, overnight) rehydration might increase viability of this fungus.
- Mix the suspension well. Use several drops (or make dilutions if desired) to inoculate the recommended solid or liquid medium, and include a control that receives no inoculum.
- Incubate the inoculum at the recommended propagation conditions. For most A. brasiliensis strains, an aerobic atmosphere at 20-30°C is usually recommended.
- Inspect for growth of the inoculum/strain regularly. Viability is noticeable typically after 1-2 days of incubation. However, the time necessary for significant growth will vary from strain to strain.
Phytophthora spp. (Phylum Oomycota)
Revival of frozen Phytophthora samples can be challenging as post-preservation viability is frequently low. Additionally, some Phytophthora species grow slowly, may be sensitive to temperature, and may be nutritionally fastidious.18 To propagate Phytophthora spp., ATCC recommends the following procedure:
- To thaw a frozen ampoule, place in a 25°C to 30°C water bath, until just thawed (approximately 5 minutes). Immerse the ampoule just deep enough to sufficiently cover the frozen material. Do not shake the ampoule.
- Immediately after thawing, wipe down ampoule with freshly prepared 70% ethanol and aseptically transfer the frozen sample to a small volume (approximately 5.0 mL) of Nutrient Broth or Yeast Mold (YM) Broth containing trace amounts of biotin and thiamine. Cultures should be incubated at the recommended temperature until growth is visible; this may take up to 20 days.
- The culture can then be transferred to moist agar media such as V8-rye medium (ATCC medium 1970), V8 juice agar (ATCC medium 343), and lima bean medium (ATCC medium 322) (See: NOTE 3).
NOTE 3:
For higher recoverability of frozen Phytophthora cells, ensure that strains are initially cultured in a nutritional broth medium prior to transfer to examining the strain on rye, V8, or lima bean based media.
Mycological Titering
Mycology cell counts are often necessary in order to monitor growth rates as well as to set up new cultures with known cell counts. Yeast and sporulating fungal cultures are commonly titered by determining the number of colony forming units per milliliter (CFU/ mL).
To enumerate CFU/mL within a suspension, at a given time-point, remove an aliquot from an actively growing solution and perform a serial dilution in an appropriate broth medium. The extent of the dilution will depend on the growth rate and phase of the strain. Each dilution should be spread-plated onto several plates consisting of an appropriate agar-based medium, then grown under optimal conditions. Following a suitable growth period, count the number of colonies that have grown on each plate. For best results, only use plates harboring colony counts within a range of 25-250 colonies as this will provide an accurate representation of the titer. To determine the CFU/mL, calculate the average of the number of colonies from one dilution series then divide by the final dilution on the plate. For example, if you have three counts of 40, 37, and 43 from a set of plates at final of dilution of 10-7, the titer would be 4.0 x 108 CFU/mL.
The growth rate and culturing requirements of yeasts and sporulating fungi can vary drastically between species, thus making it difficult to quantify a titer. ATCC recommends that plates growing sporulating fungi be read quickly as the culture can quickly grow together and individual colonies will no longer be distinguishable. For more information on how to culture a particular strain, see the product sheet for details or contact ATCC Product Experience.
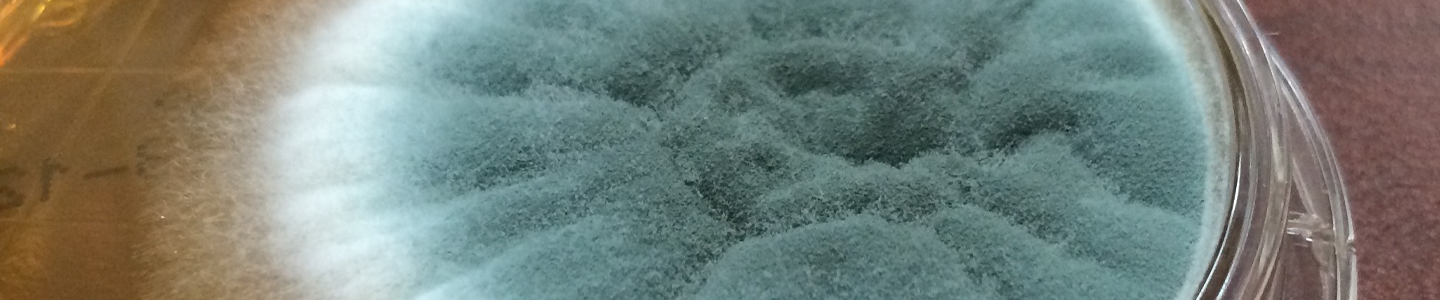
Growth Media
Mycology culture media are often mixtures of proteins, trace elements, amino acids, and carbohydrates. The requirements for these components vary among fungal and yeast strains. In addition to supplying nutrients, medium assists in the maintenance of pH. The pH can be sustained through one or more buffering systems such as MOPS or potassium phosphate. ATCC uses numerous types of media in order to provide the optimal growth conditions for each mycology strain. The recommended media for all strains is indicated on the product sheet and online at www.atcc.org.
YM Agar or Broth (ATCC medium formulation 200) is commonly used in the cultivation of yeasts, molds, and other acidophilic organisms. This medium is generated from a mixture of yeast extract, dextrose, malt extract, and peptone. YM agar and broth media provide organisms with a source of trace elements, vitamins, amino acids, carbon, and protein.
Emmons’ Modification of Sabouraud’s Agar (ATCC medium formulation 28) is used in the cultivation of pathogenic and commensal fungi and yeasts. It consists of a mixture of dextrose and neopeptone at a 2:1 ratio. This medium is a modification of Sabouraud Dextrose Agar, contains a reduced amount of dextrose, and is maintained at a neutral pH. The neutral pH enhances the recovery of some pathogenic fungi.
Potato Dextrose Agar (PDA) (ATCC medium formulation 336) is a common microbiological growth medium used to cultivate fungal and bacterial species that attack living plants or decaying plant matter. PDA is commonly generated from a filtered, boiled potato suspension mixed with dextrose and agar.
Yeast extract Peptone Dextrose (YePD) (ATCC medium formulation 1245) is a complete medium used for the cultivation of yeasts. It consists of a mixture of yeast extract, peptone, and dextrose at a ratio of 1:2:2. This medium is adjusted to a pH of 5.6.
Malt extract Agar (Blakeslee’s Formula) (ATCC medium formulation 325) is recommended for the detection, isolation, and enumeration of yeasts and molds. This medium is generated from a mixture of malt extract, glucose, and peptone.
Cornmeal Agar (ATCC medium formulation 307) is used for the cultivation of fungi and the demonstration of chlamydospore production. Cornmeal present in the agar provides fungal species with a source of carbon, nitrogen, and vitamins required for growth.
V8 Juice Agar (ATCC medium formulation 343) is recommended for sporulation and the examination of various fungal characteristics throughout the life cycle. This medium contains V8 juice supplemented with calcium carbonate.
Media Formulations
The formulations for media used by ATCC can be found online. Please note that most microbial media is not currently sold at ATCC.
Media Ingredients
Dextrose
Dextrose, also known as D-glucose, is a highly nutritious supplement used for the cultivation of fastidious microorganisms, older cultures, and cultures with small inoculums. This reagent can be used as a dextrose broth/agar medium or as a supplement within a complex or defined medium. Dextrose is predominantly used as a source of carbon.19
Peptone
Peptone is a water-soluble protein derivative used in culture media. This reagent is prepared via the partial hydrolysis of an animal protein by an enzyme or acid. Not all microbial species can use free atmospheric nitrogen. Many species require either organic or inorganic fixed nitrogen.9 Peptone is used in media as an organic source of nitrogen and is often used in serum-free medium. The nutritional value of peptone is dependent on the amino acid content that supplies the essential nitrogen. The starting material for peptone can range from animal to plant; these can include meat, soybean, casein, and whey.20 (See: NOTE 4).
Yeast Extract
Yeast extract is prepared as a water-soluble extract of autolyzed Saccharomyces cerevisiae yeast cells. During autolysis, endogenous yeast digestive enzymes break down protein content into peptides and amino acids, which can be used by fungi as a source of nitrogen. Additionally, yeast extract provides an essential source of water-soluble B-complex vitamins, carbohydrates, and free glutamic acid.21 (See: NOTE 4).
NOTE 4:
Different preparations of peptone, yeast extract, and malt extract may contain different amounts of peptides, amino acids, etc. For particularly fastidious microbes, it may be necessary to try different batches of these ingredients.
Malt Extract
Malt extract is the water-soluble portion of malted barley. This reagent is a common component in media used in the cultivation of yeasts and molds as it contains a high concentration of reduced sugars. To generate malt extract, barley is initially germinated in a process termed malting. Sugars are then extracted from the malted barley by cracking the grain in a mill then warming the barley in temperature-modulated water to induce enzyme production and subsequent sugar breakdown. The resulting substance, or “wort,” is filtered and dried for use in culture media.21 (See: NOTE 4).
Media Supplements
The growth media recommended for some fungal and yeast species may require the addition of components, such as salts and trace elements not readily available in the base medium. After supplements have been added to the base medium, the shelf life of the medium should be determined on a case-by-case basis. Media containing supplements should not be frozen as this may cause certain compounds to precipitate out of solution. Media should be stored at 2°C to 8°C. For additional information regarding the preparation, storage, or usage of specific additives, contact your local supplier or consult with the manufacturer’s product information sheet.
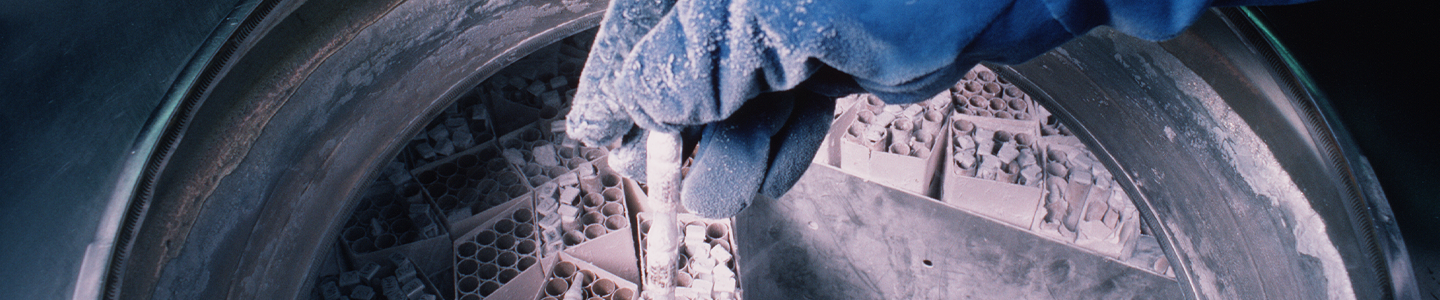
Preservation
Most yeast and fungi can be stored for many years as freeze-dried cultures (lyophilization) or at temperatures below -130°C (cryopreservation). There are many advantages of preservation which far outweigh the required investment in the equipment and supplements needed to maintain living cultures. These advantages include:
- Overall safety of stocks against loss due to equipment failure or contamination by other microbial organisms.
- Elimination of time, energy, and material costs associated with the maintenance of strains not currently in use.
- Insurance against phenotypic drift associated with prolonged passage, due to genetic instability and/ or selective pressures.
- Creating a standard working stock that can be used for a series of experiments.
Cryopreservation
Overview
Generally, freezing a suspension of living cells may result in a decrease in viability.22, 23 As the suspension is cooled below the freezing point, ice crystals begin to form and the concentration of solutes in the suspension increases. Additionally, the formation of intracellular ice crystals can result in the damage of cellular structures. This can be minimized if water within the cells is allowed to escape by osmosis during the cooling process. A slow cooling rate, generally -1°C to -10°C per minute, facilitates this progression. However, as the cells lose water, they shrink in size and will quickly lose viability if they surpass a minimum threshold volume. The addition of cryoprotectant agents, such as glycerol or dimethylsulfoxide (DMSO), will mitigate these effects.24, 25 For the preservation of mycology cultures, ATCC recommends using glycerol as a cryoprotectant.
The standard procedure for cryopreservation is to freeze material slowly until they reach a temperature below -70°C in medium that contains a cryoprotectant. Vials are then transferred to a liquid-nitrogen freezer to maintain cultures at temperatures below -130°C.
The recovery of cryopreserved cells requires the rapid thawing of the suspension in a 25-30°C water bath. The entire contents of the vial are then transferred to an appropriate growth medium.
In general, there are numerous factors that can affect the viability of recovered fungi and yeasts. These critical parameters can include the composition of the freeze material, the growth or reproductive phase of the strain, or the concentration of living material within the solution. To obtain optimal cell viability upon recovery, modify the cryopreservation procedure for each strain, being sure to harvest cultures during logarithmic phase of growth.
Contact ATCC for more information on the cryopreservation of yeasts and fungal strains. ATCC mycological strains that are frozen are commonly cryopreserved with a medium containing a final concentration of 10-20% sterile glycerol.
Freeze Medium
Glycerol and DMSO are the most common cryoprotectant agents. ATCC recommends using a 20% glycerol stock at a final concentration of 10%. However, if the strain is sensitive to glycerol, a 50% DMSO stock can be used at a final concentration of 5%. Generally, glycerol can be sterilized via autoclavation whereas DMSO must be sterilized by filtration. Care should be used when handling any DMSO solution as it will rapidly penetrate intact skin and may carry toxic contaminants along with it.
Use only reagent-grade DMSO or glycerol. Store both in aliquots protected from light. ATCC offers DMSO (ATCC 4-X) that has been thoroughly tested for use. Overall, the optimum formulations for individual yeast and fungal strains must be determined empirically.
Equipment
- Cryopreservation vials
There are two materials to choose from for cryopreservation vials: glass or plastic. Glass vials are more difficult to work with; they need to be sterilized before use, they need to be sealed with a hot flame, and they can be difficult to open. However, they are preferred for long-term storage (many years) of valuable cultures and are considered fail-safe once properly sealed. ATCC uses glass vials for the storage of seed stocks, which are placed in the lower level of a liquid nitrogen tank. Plastic, glass double-vials, and serum vials are used for the storage of distribution stocks.
If cryopreservation in glass ampoules is not possible, plastic vials can be used. Plastic vials come in two varieties: those with an internal thread and silicone gasket, and those with an external thread. Vials with an internal-thread were the first commercially available, but have some disadvantages over the external-thread version. For example, while the silicone gasket provides an excellent seal, it needs to be tightened just right; the vial will leak if the seal is too tight or too loose. - Controlled-rate freezing chambers
There are several means to achieve a cooling rate of -1°C per minute. The best method involves the use of a computer controlled, programmable electronic freezing unit (such as Thermo Scientific CryoMed Freezers), which rigorously maintains this rate of cooling. This is the method used exclusively at ATCC. Such equipment is relatively expensive and absolutely necessary for only the most sensitive strains.
A less costly approach is to place the cryopreservation vials into an insulated chamber and cool for 24 hours in a mechanical freezer at -70°C or colder. There are several commercially available freezing chambers which achieve a cooling rate very close to the ideal -1°C per minute (CoolCell LX; ATCC ACS-6000). Alternatively, the vials can be placed into a polystyrene box, with 15-mm (3/4 inch) thick walls and 1L capacity that is packed with paper, cotton wool, or foam peanuts for insulation.
Liquid Nitrogen Freezing Storage
The ultra-low temperatures (below -130°C) required for long-term storage can be maintained by specialized electric freezers, or more commonly, by liquid nitrogen freezers. There are two basic types of liquid nitrogen storage systems: immersing vials in the liquid or holding vials in the vapor phase above the liquid. The liquid-phase system holds more nitrogen and thus requires less maintenance. However, there is always a chance that some liquid will enter improperly sealed vials, which may then explode when retrieved. For this reason, ATCC strongly recommends the storage in vapor-phase.
Vapor-phase storage systems create a vertical temperature gradient within the container. The temperature in the liquid nitrogen at the bottom will be -196°C, whereas the temperature at the top will vary depending upon the amount of liquid nitrogen at the bottom and the length of time the container is opened. To ensure the safe storage of cells, maintain sufficient levels of liquid nitrogen in the container so that the temperature at the top is -130°C or colder. All storage systems should be equipped with temperature alarms.
Cryopreservation Procedure
- Incubate the yeast or fungal strain under the recommended conditions until a maximum yield is obtained.
- Add an appropriate cryoprotective agent to the suspension.
- Within a laminar flow hood, dispense 0.5 to 1.0 mL of the above mixture into sterile plastic cryovials or glass ampoules. Store the filled vials on ice prior to sealing. Glass ampoules can be sealed using a gas- oxygen torch, pulling the neck of the ampoules as it is rotated in the flame. Following sealing, disinfect glass ampoules by spraying with disinfectant.
- Place the vials into a pre-cooled (4°C), controlled-rate freeze chamber and place the chamber in a mechanical freezer at -70°C (or colder) for at least 24 hours. Alternately, use a pre-cooled (4°C) programmable freezer unit set to cool the vials at -1°C per minute until a temperature below -40°C is achieved and then set the temperature to abruptly drop to -120°C.
- Quickly transfer the vials to a liquid nitrogen or -120°C freezer. Frozen material will begin to warm up above -50°C.
- Record the location and details of the freeze.
- After 24 hours at -120°C, remove one vial, appropriately restore the strain, and determine the viability and sterility.
Recovery of Cryopreserved Cultures
The frozen solution needs to be warmed as rapidly as possible and then immediately transferred to an appropriate growth medium. Some mycology strains may take more time than normal to fully recover from cryopreservation.
- Prepare a culture vessel that contains at least 10 mL of the appropriate growth medium equilibrated for both temperature and pH.
- Remove the vial from the liquid nitrogen freezer and thaw by gentile agitation in a 25-30°C water bath (or a bath set at the normal growth temperature for that strain). Thaw the strain rapidly until all ice crystals have melted (approximately 5 minutes).
- Remove the vial from the water bath and decontaminate it by dipping in or spraying with freshly prepared 70% ethanol. Follow strict aseptic conditions in a laminar flow hood for all further manipulations.
- Unscrew the top of the vial and transfer the entire contents to the prepared growth medium.
- Examine the cultures after an appropriate length of time.
Lyophilization
Overview
Freeze-drying is a process where water and other solvents are removed from a frozen product via sublimation.26 Sublimation occurs when a frozen liquid goes directly to a gaseous state without entering a liquid phase. The freeze-drying process results in a stable, readily rehydrated product. This process consists of three steps: pre-freezing the product to form a frozen structure, primary drying to remove most water, and secondary drying to remove bound water.
During the initial freezing process, ice crystals begin to form and the concentration of solutes in the suspension increases. The method used during freezing can greatly affect the ability to freeze-dry the material. It is preferable to use slow rates of cooling as this will result in the formation of vertical ice crystal structures, thus allowing for more efficient water sublimation from the frozen product.
Freeze-dried products are hygroscopic and must be protected from moisture during storage. Additionally, these products are sensitive to changes in oxygen content and temperature, which can significantly decrease shelf life. It is important to store freeze-dried material in a manner that protects the product from exposure to moisture and oxygen, and to store the material at refrigerated temperatures (4°C).
Contact ATCC for more information on the lyophilization of yeast and fungal strains.
Equipment
- Lyophilization Vials
For the storage of lyophilized fungi and yeasts, ATCC uses sterile glass serum vials. Generally, during the lyophilization process, material is freeze-dried in a glass ampoule, which is then disinfected and sealed. - Lyophilization Apparatuses
For the lyophilization of mycology strains, ATCC employs a commercial freeze-dryer. In this freeze-drying procedure, samples are mixed with a suitable preservative, dispensed into the ampoule, and allowed to slowly freeze into a solid mass. Once frozen, samples are lyophilized within a freeze-drying system (VirTis Genesis Pilot Lyophilizer, Millrock Technology Max85 Freeze Dryer).
During the primary drying phase, water is removed from the frozen product via sublimation. This is accomplished through the use of a vacuum pump, which allows water molecules to migrate from the frozen product and condense on a moisture trap called a condenser. For this to be possible the temperature of the condenser must be colder than the product temperature; the difference in these temperatures will affect the rate of sublimation. When primary drying is complete, all residual moisture is removed by directly heating the product. During this secondary drying phase, water must be desorbed to a residual moisture content of 1% or less. This process requires a low pressure, low condenser temperature system. Once dried, the ampoules are properly sealed and stored at refrigerated temperatures (4°C).
Storage and Viability of Lyophilized Strains
Lyophilized products are hygroscopic and must be stored under moisture-free conditions. Other factors such as oxygen content and temperature can also affect the shelf-life of freeze-dried strains. Oxygen can chemically react with the product, negatively affecting culture viability. This reactivity is directly proportional to storage temperature. Therefore, lyophilized products should be stored long-term in refrigerated temperatures (4°C) under conditions that protect the sample from exposure to moisture or oxygen.
To maximize the recovery of viable cells, yeast and fungal cultures must be in optimum condition before lyophilization.27 The age of a culture can affect its ability to survive freeze-drying; for example, yeast cultures that are harvested from late log phase or early stationary phase have the greatest chance for survival during low-temperature stresses. Likewise, use of appropriate growth conditions and media, such as nutritive, non-selective growth media, during reconstitution of lyophilized strains will help to maximize recovery.28, 29
Lyophilization Procedure
- Grow the fungal or yeast strain according to recommended procedure.
- Suspend the strain in an appropriate lyophilization additive and mix thoroughly.
- In a laminar flow hood, dispense the suspension into a serum vial. Plug the ampoules loosely with slotted silicon rubber stoppers, and place the ampoules in a freeze-dryer tray. Set an acrylic plate on top of the stoppers.
- Slowly freeze the sample. Once frozen, lyophilize the sample.
- Freeze-dry the material in a commercial freeze-dryer. Hold the material at -30°C for 18-48 hours, depending on the additive. Then raise the shelf temperature by 10°C per hour until the product temperature is 25°C.
- When the cycles are complete, backfill the system with sterile nitrogen and press the caps into place. Disinfect the outside of the ampoules and return them to the laminar hood.
- Seal the vials.
Recovery of Lyophilized Cells
- To rehydrate freeze-dried strains, add 0.3 to 0.4 mL of broth or sterile water.
- Mix well and transfer to a tube containing 5 to 6 mL of broth medium.
- A few drops of this suspension may also be added to an agar slant or place. Growth medium should be chosen to maximize the recovery of cells.
- Incubate strains under the appropriate temperature and atmospheric conditions.
Preservation of Mycological Strains
Yeasts
Yeasts can be preserved by both cryopreservation and lyophilization. Below, we describe a general procedure for the preservation of yeasts.
- Grow the culture on agar.
- Suspend the cells in 20% skim milk or Reagent 18 for freeze-drying, or in 10% glycerol for freezing. Scrape the surface of the agar plate with a pipette if necessary.
Reagent 18:
0.75 g Trypticase Soy Broth
10 g sucrose
5.0 g bovine serum albumin fraction V
100 mL distilled water
Filter-sterilized through a 0.2 µm filter - Mix well and dispense 0.2 mL into each inner vial (for the double vial method) or 0.5 mL into each plastic vial for freezing.
- Freeze-dry or used a controlled-rate freeze according to general procedures.
Spore-forming Filamentous Fungi
Spore-forming filamentous fungi are often preserved by lyophilization. Sporulating strains should be grown on solid media in preparation for freeze-drying. Below, we describe a general procedure for preservation.
- Grow fungi under conditions that will produce maximum sporulation so that sufficient spores will survive the freezing and drying process. Optimum media and growth temperatures can be found on the provided product sheet.
- Prepare the lyophilization additive as a 20% solution of skim milk and store at 4°C until use. Ensure that the skim milk is cold when used.
- Prepare the spore suspension by slowly introducing about 2 mL of milk into the culture tube or plate while gently scraping the surface with the pipette. Take care to avoid raising a cloud of spores, especially with Aspergillus, Penicillium, and other dry spores. Neurospora spores are very difficult to contain; cultures for freeze-drying are usually grown on agar in 250 mL flasks.
- Transfer this suspension back into the tube containing the remainder of the skim milk and mix thoroughly. If more than one plate or tube is used, repeat the procedure for each and pool the suspension in one tube; a concentration of at least 106 spores per mL is needed.
- Many spores will begin to germinate when suspended in liquid; therefore, timing is critical when filling vials for freeze-drying. Spores should not be in the skim milk for more than two hours before being processed. Filled vials should be refrigerated while awaiting further processing.
- Dispense 0.2 mL into each vial for freeze-drying.
- Rehydrate freeze-dried fungal cultures with 0.5 to 0.9 mL of sterile water, and then place the entire contents of the vial into 6 mL of water. Allow to soak for at least 2 hours before transferring to solid media.
Filamentous Fungi
Sporulating strains are grown on solid media as for freeze-drying. Nonsporulating strains are grown either on solid or in liquid media.
Cultures with mycelium should be grown on agar within test tubes, scraped up with a pipette, and then suspended in 10% glycerol. This suspension should be dispensed in 0.5 mL aliquots into each plastic vial and preserved using a slow freeze (See: NOTE 5).
NOTE 5:
For cultures with a sticky mycelium, mycelium that will not break up, or mycelium that grows embedded in the agar, grow the culture on agar in plates. Cut out plugs containing new growth (hyphal tips) using a sterile cork borer. Place three or four plugs into each plastic freezing vial with approximately 0.4 mL of 10% glycerol, and preserve using a controlled freeze.
Special Hazards
Care must be taken during the cryopreservation or lyophilization of fungi and yeasts. Problems such as contamination, spore dispersal, breakage of glass ampoules during handling and storage, dispersal of freeze-dried materials when opening glass ampoules, and the handling of liquid nitrogen must all be considered. To prevent contamination and the dispersal of material, aseptic technique must be followed. This can include the decontamination of all equipment and vials as well as performing all preparations in a biological safety cabinet. Additionally, protective clothing should be worn during preparation to prevent contamination as well as to guard against harm due to contact with liquid nitrogen.
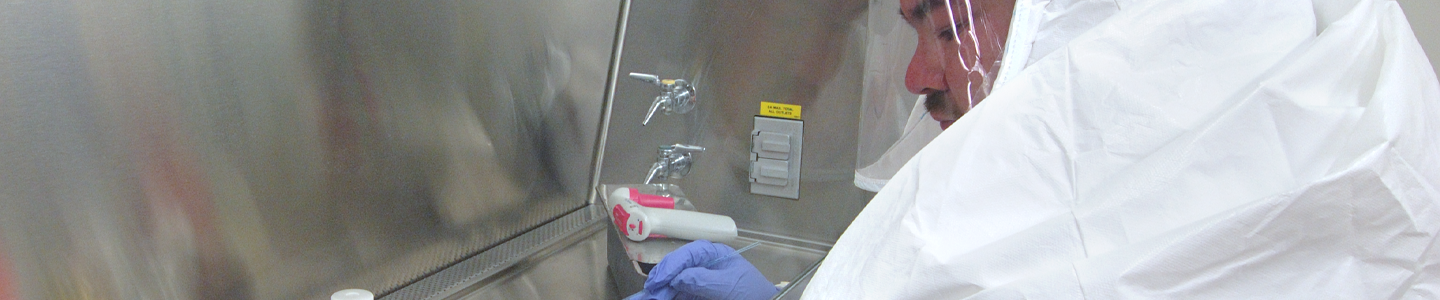
Biosafety and Disposal
Biosafety
The need for precautions when experimenting with fungus or yeast cultures depends upon the source and nature of the biological material, the experimental procedure, and the laboratory/containment conditions. Since every situation is different, the risks need to be identified for each individual strain and the appropriate precautions need to be taken before any work begins.
More information on risk assessment and precautions can be found in the CDC publication Biosafety in Microbiological and Biomedical Laboratories.30 The text of this publication is available in its entirety online (www.cdc.gov).
ATCC assigns a biosafety level (BSL) to each strain for the purposes of packaging for safe shipment. ATCC follows federal biosafety guidelines and takes several factors into consideration when assessing a potential hazard, and in some cases the ATCC assigned biosafety level is more restrictive. Generally, ATCC only ships and stores mycology strains with a biosafety level assignment of 1, 2, or 3.
Biosafety Level 1
- Work involving well-characterized strains not known to consistently cause disease in immunocompetent adult humans.
- Work can be conducted on the bench top using aseptic technique, no special containment equipment or facility is required.
Biosafety Level 2
- Work involving strains that pose a moderate hazard to healthy adult humans.
- Work should be conducted in designated biological safety cabinets within laboratories with restricted access.
Biosafety Level 3
- Work involving indigenous or exotic agents that may cause serious or potentially lethal disease via inhalation.
- Work should be conducted in biological safety cabinets localized inside a specialized BSL-3 containment facility within laboratories with restricted access.
As the recipient of yeasts or fungi, take into account not only the nature of the material but also the manipulations employed during its handling when assessing the potential laboratory risk. Keep in mind that there will be situations where the intended use of an agent may require more stringent precautions than associated with the assigned biosafety level.30
Disposal of Infectious Materials
All cultures, stocks, and potentially infectious materials need to be properly decontaminated prior to disposal. The written method for proper decontamination should be available in the laboratory and BSL facility. Several methods of sterilization include chemical disinfection, incineration, use of an autoclave, or any other validated decontamination method. More information on the disposal of mycology cultures can be found in the Center for Disease Control (CDC) publication Biosafety in Microbiological and Biomedical Laboratories.30
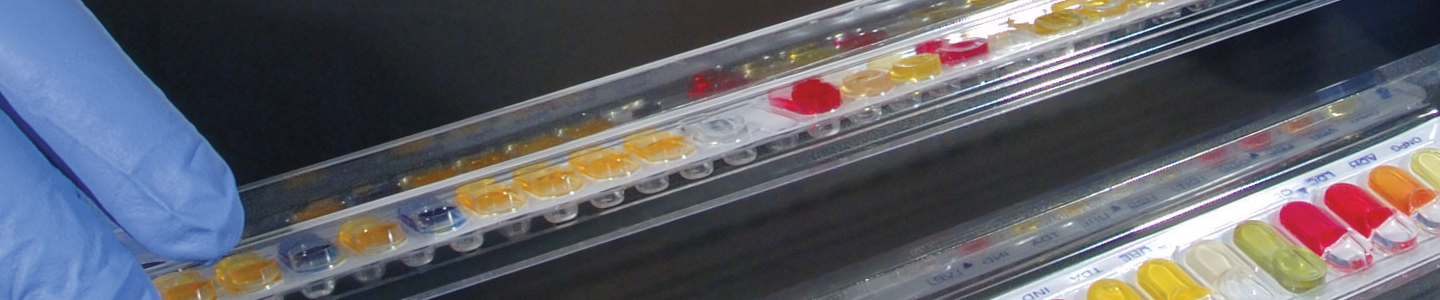
Mycological Authentication
When preparing yeasts or fungi for distribution, ATCC performs numerous quality control (QC) assays to guarantee that the product is of the highest standard before it reaches the consumer. All strains undergo thorough phenotypic and genotypic examinations to ensure that strain identification is accurate, the culture is pure, and that all biochemical results are consistent. Described below are several tests that ATCC commonly performs on yeast and fungal strains during QC analysis.
Phenotypic Characterization
When yeast and fungal strains are received by ATCC, they are analyzed for characteristic phenotypic traits associated with the species. Features commonly examined for filamentous fungi include morphological structures, such as fruiting bodies and mycelia, and variations in life stages, such as the presence or absence of a yeast stage. Mycelia typically consist of microscopic, tubular hyphae that branch out in multiple directions toward various substrates through apical growth. These filaments constitute the body of a fungus and are commonly interrupted by partitions called septa. The dispersal and variations of septa differ among species and can be used as part of the morphological authentication process. In addition to the analysis of hyphal structure, fungi authentication requires the analysis of fruiting bodies. These reproductive structures are spore-producing, multicellular structures known as the sporocarp. Fruiting bodies form during the sexual phase of the fungal life cycle, and can range in morphology among species. The stage of fungal reproduction, to include the asexual anamorph stage or the sexual teleomorph stage, is also studied for fungal identification.
In the analysis of fungal strains such as ascomycetes and basidiomycetes, many of which exist as yeasts throughout most of their life cycles, ATCC scientists observe morphology, reproduction, and the ability to assimilate and ferment various carbohydrates. Generally, yeasts exist as single celled organisms. Some species, however, can form multicellular structures through the formation of septate mycelium or pseudohyphae, which are budding yeasts that remain joined. The formation or absence of these complex structures can often be indicative of the type of fungus and can be used as part of the species authentication process. In addition to these structures, yeast identity is authenticated through the type of reproduction observed, either asexual or sexual. In asexual reproduction, yeasts form daughter cells through mitosis in a process referred to as fission or budding. In contrast, haploid yeast cells are able to reproduce through sexual reproduction. In this latter form of reproduction, opposite types of haploid yeast cell first undergo shmooing, then subsequently fuse together to create a diploid cell. The resulting diploid cell then buds and eventually forms a colony of diploid yeast cells. Lastly, yeast identity is confirmed through the examination of carbohydrate assimilation and fermentation studies. This can be analyzed using the API 20C System, the Uni-Yeast Tek System, and the VITEK Yeast Biochemical Card.
Genotypic Characterization
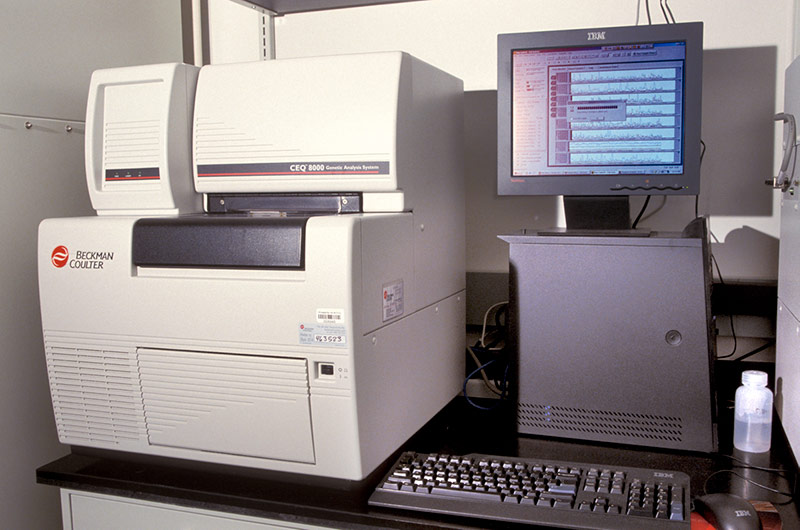
ATCC genotypically confirms yeast and fungal species through sequencing regions of both the large and small ribosomal subunits as well as the internal transcribed spacer regions connecting the two subunits. Specifically, the internal transcribed spacers ITS1 and ITS2, the 18S rRNA gene from the small subunit, and both the 5.8S rRNA gene and 5’ end of the 28S rRNA gene (D1/D2 hyper-variable region) from the large subunit, are analyzed for species specific sequences. These sequences are commonly used to analyze yeast and fungal phylogeny and taxonomy as they are highly conserved among mycology species.
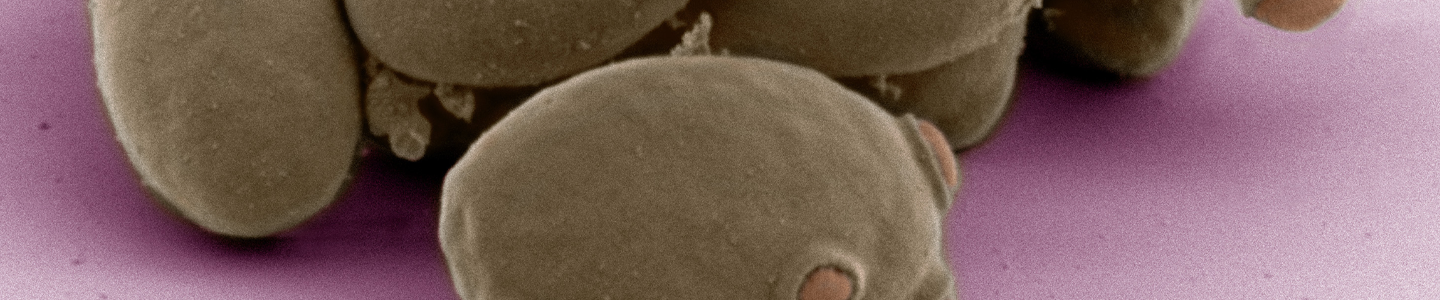
Mycological Applications
For centuries, yeasts and fungi have been used as food products or for the production of consumable products via fermentation. In recent years mycology strains have also been commercialized for use in biomedical and pharmaceutical analysis, antibiotic production, biofuel production, biocontrol, and bioremediation. Below, we further describe these various applications.
Biomedical and Pharmaceutical Standards
Over the last several decades, fungi and yeasts have emerged as a major cause of nosocomial mycoses among immunocompromised individuals in the United States.31 Existing therapies necessitate the use of antifungal drugs. However, due to both natural and societal selective pressures, the number of antifungal drug-resistant strains has been steadily increasing. For the development and advancement of novel therapeutic strategies, biomedical and pharmaceutical research is required. To aid in this research, pathogenic strains of fungi and yeasts can be used as reference standards to determine drug efficacy and required potency. Clinical strains with common antifungal resistant phenotypes are particularly useful in the drug discovery as these would likely be the cause of most nosocomial infections.
Consumable products
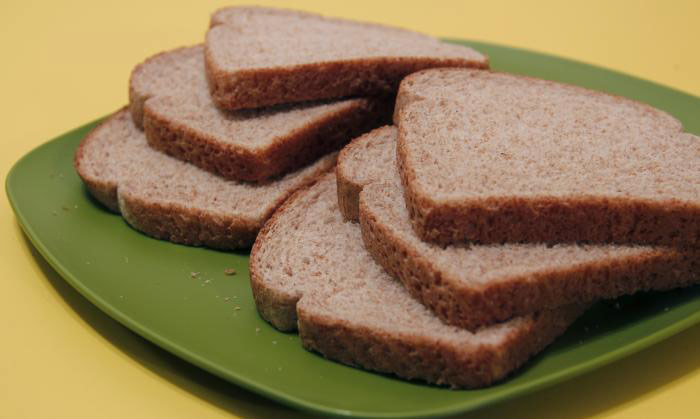
Image of bread courtesy of Debora Cartangena, CDC
Yeast and fungal strains are commonly used as or in the production of various consumable products including mushrooms, yeast extract, bread, beer, wine, bleu cheese, and soy sauce. In the production of breads, beer, and wine, various species of Saccharomyces, among other genera, are commonly used to convert fermentable sugars into ethanol and carbon dioxide. The flavor and consistency of these products are affected by the strain of yeast used. Bleu cheeses are commonly produced from cow’s milk, sheep’s milk, or goat’s milk cheeses that have cultures of the mold, Penicillium roqueforti or Penicillium glaucum. These soft cheeses are commonly named for the location they were produced in, such as Roquefort cheese in France, or Gorgonzola in Italy. Lastly, soy sauce is prepared from the fermented paste of boiled soybeans, roasted grain, brine, and Aspergillus oryzae or Aspergillus sojae molds. This latter food product is commonly used as a condiment or as a traditional ingredient in East and Southeast Asian cuisines.
Antibiotics
Antibiotics are compounds used to either destroy or impede the growth of bacterial or fungal microorganisms via a variety of mechanisms, such as the inhibition of cell wall, protein, or DNA synthesis. Many currently known fungal-produced antibiotics are derived from byproducts generated by species within the genera Penicillium and Acremonium. These genera are known to produce beta-lactams, a broad class of antibiotics recognized for their characteristic ring structure. This group comprises a variety of penicillin derivatives, cephalosporins, and carbapenems. Penicillin derivatives such as penicillin G, ampicillin, and amoxicillin are commonly derived from Penicillium fungi. Cephalosporins, such as cephalexin, cefaclor, and cefixime, are commonly derived from the fungus Acremonium. Fungal strains are believed to produce antibiotics as a means to diminish or eradicate the growth of other invading species that may be either harmful or in competition for a similar food source. Within human society, antibiotic production is critical for the treatment of infectious diseases and is often used agriculturally in the biological control of plant pathogenic microorganisms.
Biofuels
Currently most of our energy is supplied through petroleum, coal, and natural gas deposits. These sources of energy, however, are both finite and non-renewable. Recently, efforts have been made to generate sustainable energy via the production of biofuels from commercially available products such as corn and sugar cane. However, these novel sources of energy require food to be used as fuel rather than for human sustenance. To resolve these aforementioned issues, current biofuel research is focusing on the use of fungi in the catabolism of cellulose into desired hydrocarbon fuel compounds. The goal is to discover fungal strains that have metabolic pathways that can generate biofuels through the degradation of biowastes or weeds, thus creating a sustainable form of energy without diminishing the food supply.
Biological Control
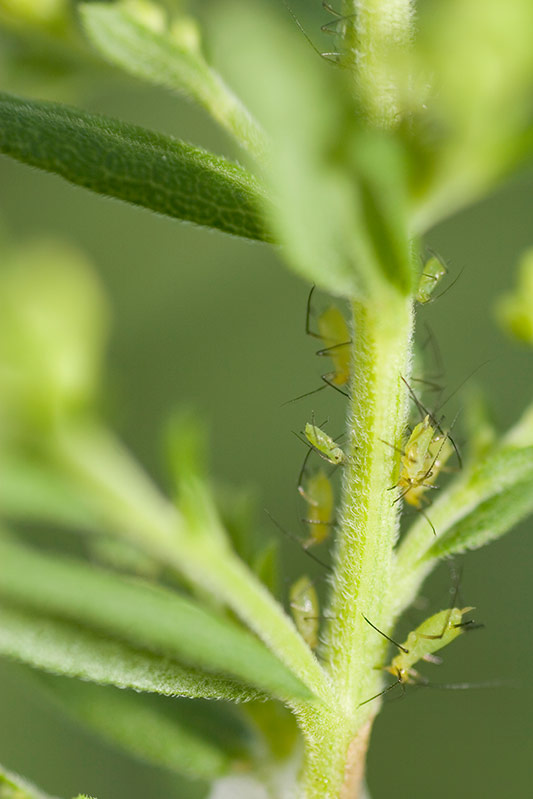
Biological control is a method that uses living organisms to control agricultural pests, such as insects or plant pathogens. It is seen as an environmentally friendly means of pest control because it leaves behind no chemical residues that may be harmful to humans or other organisms. However, specificity is a major concern in biological control; although biological control agents can be selective against a particular pest species, there is a risk that the control agent may also attack desirable species. Different fungal species have been used in the control of pests, weeds, and diseases through mechanisms such as competitive exclusion, mycoparasitism, or the production of metabolites. Beauveria bassiana, Paecilomyces fumosoroseus, and Lecanicillium spp., for example, are entomopathogenic fungi used in controlling different insect populations such as aphids, thrips, and white flies. Other fungal species, including those within the Trichoderma genera, are used to manage certain plant pathogens such as Dutch Elm disease or Silver Leaf disease.
Bioremediation
Bioremediation is the use of the metabolic pathways of microorganisms to remove pollutants from the environment. Fungal-based bioremediation processes, termed mycoremediation, have been used in recent decades in the degradation of hazardous chemicals such as xenobiotics, creosote, and paper mill wastes. The ability of fungal species to breakdown wastes is due to the secretion of a variety of extracellular enzymes. Fungal decomposition of lignin and cellulose, in particular, is not only a key metabolic process in the carbon cycle,32 but aids in the decomposition of paper-based waste products such as pulp or paper mill byproducts. Moreover, lignin-degrading fungi, such as Phanerochaete chrysosporium, P. sordida, and Tramates hirsuta, have been implicated in the degradation of pentachlorophenyl (PCP) and creosote in soil.33 In addition to its role in PCP and creosote degradation, P. chrysosporium has been shown to degrade a number of toxic xenobiotics, including aromatic hydrocarbons, chlorinated organics, and nitrogen aromatics.34
Download a PDF of our mycology guide
Download now
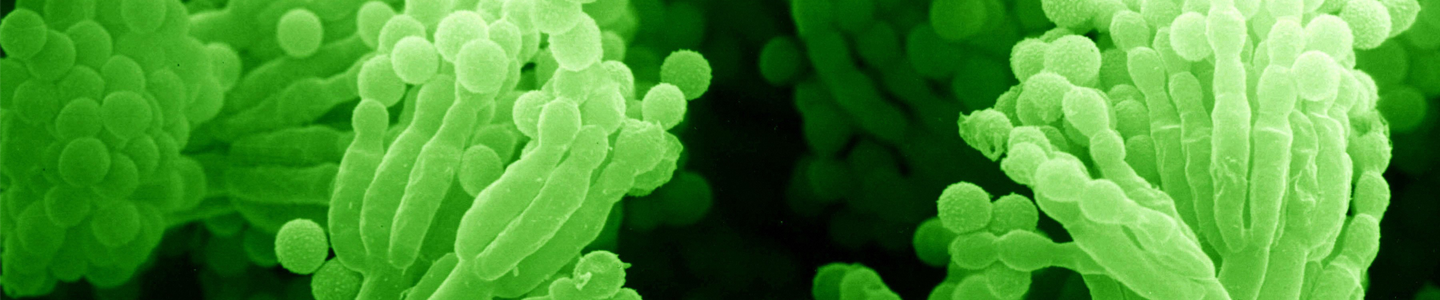
Glossary
Acervulus (pl. acervuli). A mat of conidiogenous cells which breaks through the epidermis of the host to release spores.
Achlorophyllous. Lacking chlorophyll.
Anamorph. An asexual reproductive stage in some fungal species.
Annellide. A conidiogenous cell that produces a series of ring-like scars at the conidiogenous apex.
Annulus (pl. Annuli). A ring on the stem of certain mushrooms.
Antheridium (pl. Antheridia). A male sex organ. It attaches to the female oogonium during mating of oomycetes. The same term is applied to the male sex organ in ascomycetes.
Apothecium (pl. Apothecia). The fruiting body of a cup fungus (discomycete).
Appressorium (pl. Appressoria). An expanded or flattened tip of a hypha which attaches to the epidermis of a host.
Arbuscular mycorrhiza. A type of fungal mycorrhiza in which the fungus penetrates the cortical cells of the roots of a vascular plant.
Arbuscules. Treelike, hyphal branches produced within host cells by endomycorrhizal fungi.
Arthrospore. A spore resulting from the fragmentation of a hypha.
Ascocarp (pl. Ascomata). The fruiting body of an ascomycete.
Ascostroma (pl. Ascostromata). A stromatic ascocarp bearing asci directly in locules within the stroma.
Ascus (pl. Asci). The spore sack of an ascomycete.
Axenic. A pure culture, existing without another culture present.
Basidiocarp (pl. Basidiomata). The fruiting body of a basidiomycete.
Basidiospore. Spores produced by members of the phylum Basidiomycota.
Basidium (pl. Basidia). The spore-producing structure of a basidiomycete.
Capillitium (pl. Capillitia). Thread-like structures among the spores of gasteromycetes and myxomycetes.
Chlamydospore. A thick-walled spore, formed inside a hypha, which functions as a resting spore.
Cleistothecium (pl. Cleistothecia). An enclosed, small fruiting body of several kinds of ascomycetes.
Coenocyte. An organism made up of a multinucleate, continuous mass of protoplasm enclosed by one cell wall.
Columella (pl. Columellae). A sterile structure at the top of the stalk of a sporangium.
Conidiophore. A simple or branched hypha arising from a somatic hypha. These structures bear one or more conidiogenous cells at either its tip or side.
Conidium (pl. Conidia). A nonmotile, asexual spore.
Enteroblastic. A form of conidiogenesis in which the existing inner wall or neither wall layer of the conidiogenous cell is used in the formation of conidia.
Entomopathogen. An organism that can act as a parasite of insects resulting in either death or a disabling disease.
Excipulum (pl. excipula). The outer layer of an apothecium.
Flagellum (pl. Flagella). A hair, whip, or tinsel-like organelle used to propel a motile cell.
Fruiting body. The part of a fungus from which spores are released.
Gametangium (pl. Gametangia). A structure that contains gametes.
Haustorium (pl. Haustoria). A fungal organ which penetrates a host and absorbs nutrients.
Heterogametangic. Organisms whose male and female gametes are morphologically distinguishable.
Heterokont. A biflagellate condition where the flagella are unequal in length, movement, or form.
Heterotroph. An organism that cannot fix carbon and uses organic sources of carbon for growth.
Holoblastic. A form of conidiogenesis in which all cell walls are incorporated into the new conidium.
Hydrogenosome. A membrane-enclosed organelle found in some anaerobic organisms, including fungi, trichomonads, and ciliates. These organelles take the place of energy-producing mitochondria, producing molecular hydrogen as a byproduct of energy generation under anaerobic conditions.
Hypha (pl. Hyphae). A part of the vegetative portion of a fungus that resembles thin threadlike structures. These structures are marked by septa at each junction.
Macrocyst. A thick-walled spore which is the sexual stage of Acrasiomycetes.
Merosporangium (pl. Merosporangia). A cylindrical sporangium characteristic of several genera in the Mucorales.
Mitosome. An organelle found in some unicellular eukaryotic organisms. This organelle is commonly found in anaerobic or microaerophilic organisms that lack mitochondria.
Monoblastic. Only one conidiogenous site per holoblastic conidiogenous cell.
Monotretic. Only one conidiogenous site per tretic conidiogenous cell.
Mycelium (pl. Mycelia). The main portion of a fungus that consists of a loose network of the feeding and reproducing hyphae.
Mycorrhiza (pl. Mycorrhizae). A symbiotic relationship between the hyphae of certain fungal species and the absorptive organs of plants. This relationship is primarily associated with the plant root system.
Nosocomial. Originating in a hospital, often referring to a disease or infection.
Oogonium (pl. Oogonia). The female sex organ in the oomycetes.
Oospore. The sexually produced spore of the oomycetes.
Operculum (pl. Opercula). The opening on a sporangium or ascus from which spores emerge.
Ostiole. The opening of a pycnidium, perithecium, or pseudthecium.
Paraphyses (sing. Paraphysis). Sterile, basally attached thread-like elements inside the hymenium of perithecia and apothecia.
Percurrent. Conidia are formed repeatedly at the same locus, producing a series of ring-like extensions.
Periphyses (sing. Periphysis). Short thread-like elements that form a fringe lining the inside of an ostiole or of a pore in a stroma.
Perithecium (pl. Perithecia). Small, sack-like fruiting body of several types of ascomycetes.
Phialide. A condiogenous cell which produces conidia in basipetal fashion and extrudes them from an opening in the cell.
Pileus (pl. Pilei). The cap of a mushroom.
Plasmodiocarp. The branched, vein-like fruiting structure of many myxomycetes.
Polyblastic. Several conidiogenous sites per holoblastic conidiogenous cell.
Polyphialides. Phialides which have more than one conidiogenous locus.
Polytretic. Several conidiogenous sidtes per tretic conidiogenous cell.
Progametangium (pl. Progametangia). A cell that gives rise to a structure, termed gametangium, that contains gametes.
Pseudohypha (pl. Pseudohyphae). A chain of fungal cells that is intermediate between a chain of budding yeast cells and a true hypha. Unlike hyphae, junctions are marked by constrictions rather than septa.
Pseudoparaphyses (sing. Pseudoparaphysis). Wide, septate, sterile threads attached to the roof and base of an ascostroma.
Pseudothecium (pl. Pseudothecia). The sack-like fruiting structure of a loculoascomycete.
Psycrophile. An organism capable of survival in extremely cold temperatures.
Pycnidium (pl. Pycnidia). A hollow conidioma lined with conidiophores.
Pycnium (pl. Pycnia). The spermogonium of the rust fungi.
Reniform. Kidney or bean shaped.
Rhizoid. A root-like structure at the base of a thallus.
Saprobe. An organism that uses decaying organic matter for nutrition.
Sclerotium (pl. Sclerotia). A small, hard, multicellular, resting body which can germinate.
Septum (pl Septa). Internal cross-walls that divide hyphae. Septa are usually perforated by pores to allow ribosomes and mitochondria to flow between cells.
Seta (pl. Setae). A hair-like bristle.
Shmooing. A process in which sexually reproducing haploid yeast cells become longer and thinner in preparation for joining. Once elongated, opposite haploid type cells are able to fuse and join their nuclei together to create a diploid cell.
Sporangiolum (pl. Sporangiola). A small sporangium containing few spores.
Sporangiophore. The hypha that bears a sporangium.
Sporangiospores. Spores that are produced in a sporangium.
Sporangium (pl. Sporangia). A spore-containing, sack-like body on the tip of a sporangiophore.
Sporic Meiosis. A haplodiplonic life cycle. Mitotic division occurs in both the haploid and diploid phases. The zygote divides mitotically to produce a diploid sporophyte. The sporophyte creates spores via meiosis which then divide mitotically to produce haploid individuals called gametophytes. The gametophytes produce gametes by mitosis.
Sporocarp. The fruiting body of a fungus.
Sporodochium (pl. Sporodochia). A cushion-shaped stroma covered with condiogenous cells.
Sporoplasm. The infectious protoplasmic material of a spore.
Sterigma (pl. Sterigmata). A small hyphal branch which bears a conidium, basidiospore or sporangium.
Stipe. The stalk of a mushroom.
Stroma (pl. Stromata). A compact body on which fruiting bodies are produced.
Subiculum (pl. Subicula). A loose hyphal network in which fruiting bodies are formed.
Sympodial. The main axis of a conidiophore that elongates in a zig-zag manner.
Synnema (pl. Synnemata). A group of conidiophores closely packed together to form a single, spore-bearing stalk.
Teleomorph. A sexual reproductive stage in some fungal species.
Teliospore. The sexual spore of a rust or smut fungus.
Thallic. The form of conidiogenesis in which conidia are produced by fragmentation of a hypha.
Thallus (pl. thalli). The body of a fungus or plant.
Thermophile. An organism that is capable of surviving in extremely warm temperatures.
Tretic. Conidia produced by protrusion of the inner wall through pores in the outer wall.
Zoosporangium (pl. Zoosporangia). A sporangium that contains zoospores.
Zoospore. A motile spore with one or more flagella.
Zygosporangium (pl. Zygosporangia). A sporangium containing a zygospore.
Zygospore. The sexual spore of a zygomycete.
Zygotic Meiosis. A haplontic life cycle. Meiosis of a zygote immediately after the fusion of two cell nuclei. This ends its diploid phase and produces several haploid cells. These cells then divide mitotically to form either multicellular individuals or more haploid cells.
References
- Alexopoulos, C.J., Mims, C.W. & Blackwell, M. Introductory Mycology (ed. Harris, D.) (John Wiley & Sons, Inc., 1996).
- Hawksworth, D.L. The magnitude of fungal diversity: the 1.5 million species estimate revisited. Mycol. Res. 105, 1422-1432 (2001).
- Ruiz-Herrera, J. Fungal Cell Wall: Structure, Synthesis and Assembly (CRC Press, Boca Raton, FL, 1992).
- Powell, M.J. Looking at Mycology with a Janus Face: A glimpse at Chytridiomycetes Active in the Environment. Mycologica 85, 1-20 (1993).
- Hempel, S., Renker, C. & Buscot, F. Differences in the species composition of arbuscular mycorrhizal fungi in spore, root and soil communities in a grassland ecosystem. Environ Microbiol 9, 1930-8 (2007).
- Kuhn, G., Hijri, M. & Sanders, I.R. Evidence for the evolution of multiple genomes in arbuscular mycorrhizal fungi. Nature 414, 745-8 (2001).
- Agrios, G.N. Plant Pathology (Academic Press, San Diego, 1988).
- Kohlmeyer, J. & Kohlmeyer, E. Marine Mycology- The higher Fungi (Academic Press, New York, 1979).
- Bakkeren, G., Kamper, J. & Schirawski, J. Sex in smut fungi: Structure, function and evolution of mating-type complexes. Fungal Genet Biol 45 Suppl 1, S15-21 (2008).
- James, T.Y. et al. A molecular phylogeny of the flagellated fungi (Chytridiomycota) and description of a new phylum (Blastocladiomycota). Mycologia 98, 860-71 (2006).
- James, T.Y. et al. Reconstructing the early evolution of Fungi using a six-gene phylogeny. Nature 443, 818-22 (2006).
- Akin, D.E. & Borneman, W.S. Role of rumen fungi in fiber degradation. J Dairy Sci 73, 3023-32 (1990).
- Hibbett, D.S. et al. A higher-level phylogenetic classification of the Fungi. Mycol Res 111, 509-47 (2007).
- van der Giezen, M. et al. Conserved properties of hydrogenosomal and mitochondrial ADP/ATP carriers: a common origin for both organelles. EMBO J 21, 572-9 (2002).
- Ironside, J.E. Multiple losses of sex within a single genus of Microsporidia. BMC Evol Biol 7, 48 (2007).
- Sumbali, G. The Fungi (ed. Johri, B.M.) (Alpha Science, Middlesex, 2005).
- Dick, M.W. in Handbook of Protoctista (eds. Margulis, L., Corliss, J.O., Melkonian, M. & Chapmann, D.J.) (Jones and Bartlett, Boston, 1990).
- Houseknecht, J.L., Suh, S.O. & Zhou, J.J. Viability of fastidious Phytophthora following different cryopreservation treatments. Fungal Biol 116, 1081-9.
- Versalovic, J. Manual of Clinical Microbiology (American Society for Microbiology, Washington DC, 2011).
- Convention, T.U.S.P. The United States Pharmacopeia 31- The national formulary 26 (The United States Pharmacopeial Convention, Inc., Rockville, MD, 2008).
- Diagnostics, B. Difco & BBL Manual, Manual of Microbiological Culture media (Copyright by Becton Dickinson and Company, Sparks, MD, 2009).
- Mazur, P. The role of intracellular freezing in the death of cells cooled at supraoptimal rates. Cryobiology 14, 251-72 (1977).
- Mazur, P. Cryobiology: the freezing of biological systems. Science 168, 939-49 (1970).
- Fahy, G.M. The relevance of cryoprotectant "toxicity" to cryobiology. Cryobiology 23, 1-13 (1986).
- Meryman, H.T. Cryoprotective agents. Cryobiology 8, 173-83 (1971).
- Rowe, T.W. Optimization in freeze-drying. Dev Biol Stand 36, 79-97 (1976).
- Heckly, R. Principles of preserving bacteria by freeze-drying. Dev Ind Microbiol 26, 379-395 (1985).
- Ray, B. Methods to detect stressed microorganisms. J. Food Prot. 42, 346-355 (1979).
- Miyamoto-Shinohara, Y. et al. Survival rate of microbes after freeze-drying and long-term storage. Cryobiology 41, 251-255 (2000).
- United States Department of Health and Human Serves, C.f.D.C., and National Institutes of Health. Biosafety in Microbiological and Biomedical Laboratories (U.S. Government Printing Office, HHS Publication No. (CDC) 21-1112, Washington DC, 2009).
- Moudgal, V. & Sobel, J. Antifungals to treat Candida albicans. Expert Opin Pharmacother 11, 2037-48.
- Bennett, J.W. & Faison, B.D. Use of Fungi in Biodegradation (Washington, 1997).
- Lamar, R.T., Evans, J.W. & Glaser, J. Solid phase treatment of pentachlorophenol-contaminated soil using lignin degrading fungi. Environ. Sci. Technol. 27, 2566-2571 (1993).
- Barr, D.P. & Aust, S.D. Mechanisms the white rot fungi use to degrade pollutants. Environ. Sci. Technol. 27, 79A-87A (1994).