ATCC's toxicology products are at the forefront of advancing scientific research and ensuring safety in various industries. With a comprehensive collection of authenticated cell lines, growth reagents, and cell viability assays, ATCC provides researchers with the reliable tools needed for standardized and reproducible toxicology studies.
Item 17/100: The Best Cell Type for In Vitro Liver Models
Sujoy Lahiri, PhD, Lead Scientist
ATCC® PCS-450-011™ – HepatoXcell™ – Primary Human Hepatocytes and Hepatocyte Media Systems
It is estimated that more than one quarter of the global population is affected by non-alcoholic fatty liver disease (NAFLD).1 However, most of the people affected by NAFLD remain oblivious as the disease usually manifests at an advanced irreversible stage of non-alcoholic steatohepatitis (NASH). Thus, fatty liver disease has been termed as a silent epidemic affecting more people at any time point than any other illness.2
For the treatment of fatty liver and many other liver diseases (e.g., hepatitis) as well as the study of drug metabolism, drug uptake, or liver toxicity of various pharmaceutical and chemical compounds, the best in vitro model is built using primary human hepatocytes. Hepatocytes are the major cell type of liver and are responsible for most of the functions the liver is attributed for, such as the metabolism of food and drugs, synthesis of urea and albumin, removal of toxins, and production of cholesterol.3 Hence, hepatocytes isolated from the human liver represent the liver functions more accurately than any other cell type and are the gold standard for in vitro liver modeling.4
ATCC now provides HepatoXcell™ primary human hepatocytes for use in disease research and drug discovery. Currently, the HepatoXcell™ portfolio offers three different types of primary hepatocytes based on their plate-ability: HepatoXcell™ Pro and Plus cells can be cultured for 7 and 3 days, respectively, and HepatoXcell™ Eco are suitable for suspension culture. To support the growth and maintenance of HepatoXcell™ primary human hepatocytes, ATCC also offers three different hepatocyte media: HepatoXcell™ Thawing Medium helps to enrich viable cells after thawing the cryopreserved hepatocytes, and HepatoXcell™ Plating Medium and Maintenance Medium enable hepatocyte initiation and maintenance, respectively. Researchers around the globe are now able to use ATCC’s HepatoXcell™ hepatocytes for their in vitro hepatic modeling.
Item 18/100: Revolutionizing Toxicology with iPSCs
Carolina Lucchesi, PhD, Principal Scientist
ATCC® ACS-1028™ – ATCC-BXS0114 Human [African American Female] Induced Pluripotent Stem (IPS) Cells
Induced pluripotent stem cells (iPSCs) are transforming toxicology by providing a versatile and human-relevant platform for assessing the safety of new drugs and chemicals. iPSCs can be derived from adult cells and reprogrammed to differentiate into various tissue types, including lung, liver, neuronal, and cardiac tissues.5 ATCC® ACS-1028™ cells were used in a recent groundbreaking study that demonstrated the development of cardioids.6 These cardiac organoids provided a sophisticated model for studying cardiotoxicity, offering insights into the electro-metabolic interactions within human heart tissue. The findings from this study highlight the potential of iPSCs to revolutionize toxicology by providing more accurate and human-relevant data.7 This ability to generate patient-specific cells makes iPSCs invaluable for personalized toxicology studies, allowing researchers to predict individual responses to substances more accurately.7 Overall, high-quality iPSCs are essential for advancing toxicology research and improving the predictive power of in vitro models.5 By using iPSCs, scientists can create more physiologically relevant models that better mimic human responses, reducing the reliance on animal testing and enhancing the efficiency of the drug development process.6
Item 19/100: Neuroblastoma Cells and their role in Neurotoxicity Research
Robert Brown, PhD, Senior Biologist
ATCC® CRL-2266™ – SH-SY5Y
In vitro screening for neurotoxicity requires cell models that effectively mimic central nervous system (CNS) cell types, particularly neuronal cells. A mainstay of neurotoxicity research since their development,8 SH-SY5Y neuroblastoma cells are the result of sequential subcloning of the parental neuroblastoma line SK-N-SH, performed by June Biedler and Barbara Spangler at Memorial Sloan Kettering.9 The parental SK-N-SH cells exhibit a dual phenotype of adherent, epithelial-like cells (termed substrate adherent or “S-type”) and semi-adherent, neuroblast-like cells (termed “N-type”) with cells spontaneously interconverting between the two.10 SH-SY5Y cells were generated by sequential subcloning of neuroblast-like population of SK-N-SH, resulting in a cell line enriched for the neuroblast phenotype (though some S-type remain due to interconversion). As a consequence of this enrichment, SH-SY5Y cells show corresponding increases in the catecholaminergic enzymes Tyrosine Hydroxylase (TH) and Dopamine Beta-Hydroxylase (DBH) as compared to SK-N-SH, mimicking a phenotype of catecholaminergic neuroblasts.
The utility of SH-SY5Y cells in neurotoxicity research is underlain by their noteworthy ability to differentiate to neuron-like cells. When undifferentiated, these cells resemble immature catecholaminergic neuronal cells, producing the neurotransmitters dopamine and norepinephrine as well as a variety of associated neuroblast and immature neuronal markers.11 While undifferentiated SH-SY5Y cells can and have been used in neurotoxicity studies, they are often differentiated to more mature neuronal phenotypes by the presence of various media additives, most commonly retinoic acid.12 After differentiation, SH-SY5Y cells demonstrate significant neurite outgrowth, apparent synapse formation, and expression of many mature neuronal markers (Tau, NF-H, MAP2, TUJ1, and NeuN).13,14 Depending on the specific protocol used, these cells can be differentiated towards adrenergic,15 dopaminergic,16 glutamatergic,17 and cholinergic18 neuronal phenotypes, enabling their use in a wide array of disease models, developmental studies, and drug screens. As a result, these cells are a powerful tool to study CNS cellular responses in both their undifferentiated and differentiated states.
Item 20/100: Immortalized Normal Lung Epithelial Cells
Chuck Na, MS, Innovation Manager
ATCC® CRL-4011™ – NuLi-1
One of the greatest gifts to scientific research is the donation of organs by patients. In addition to the direct life-saving transplantation field, donated organs are used to develop essential tools like immortalized cell lines that are used in the research and development of new treatments and diagnostics and in gaining a deeper understanding of cellular biology.
At the end of the 20th century, researchers working in the University of Iowa Microbiology and Immunology department worked with donated lungs to study normal and cystic fibrosis human airway epithelial (HAE) cells.19 The group, led by Dr. Aloysius J. Klingelhutz, isolated HAE primary cells from a normal lung from a 36-year-old patient and introduced the genes hTERT and HPV-16 E6/E7 to immortalize the cell lines; without the two introduced genes, the primary cells are not capable of continuous culturing. The developed cell line, named NuLi-1, exhibited an extended lifespan beyond 30 passages without any slowdown of growth. Importantly, the NuLi-1 cells retained processes like developing cilia and keeping normal sodium and chloride ion transport. Immortalized cell lines like NuLi-1 allow scientists to use a reliable, continuous supply of research materials that can reliably form lung structures to study ion transport, airway epithelial mucus production, immunology response, and disease progression of viral and bacterial infections to the lung.
Recent published studies have used NuLi-1 to study the efficacy and toxicology of azithromycin to treat cystic fibrosis inflammation,20 explore mitochondrial impact in cystic fibrosis,21 and generate a wound repair dataset under high-throughput drug screening experimental conditions for AI-driven cell tracking.22
Item 21/100: The Superstar In Vitro Model for Intestinal Insights
Meghan Sikes, MS, Senior Biologist
ATCC® HTB-37™ – Caco-2 [Caco2]
In 1977 at the Sloan-Kettering Institute for Cancer Research, researcher Jorgen Fogh developed an immortalized human colon cancer cell line known as Caco-2.23 Fogh deposited this tumorigenic cell line with ATCC under catalog number ATCC® HTB-37™; since that time, this cell line has been found to be an ideal model system for intestinal permeability and toxicology studies. Prior to the 1980’s, models to understand epithelial permeability in the intestine employed intestinal loops and other systems that didn’t perfectly mimic the real environment of the intestinal mucosa. In 1989, Hidalgo and his colleagues found that Caco-2 cells from ATCC were permeable and could be functionally polarized to realistically mimic the usual apical-basal polarity of intestinal epithelia, making the cell line a valuable in vitro model.24
Even to this day, Caco-2 cells remain a critical cell line for toxicology evaluations due to their intestinal absorption modeling capabilities.25 For instance, Caco-2 cell lines are frequently used for colorimetric MTT tests, where a mono-tetrazolium salt reagent can pass through cellular membranes and is reduced by metabolically active cells. This assay has been used for decades to determine appropriate concentrations of substances, measure drug cytotoxicity, and monitor cell viability.26,27 MTT tests on Caco-2 cells have been useful in determining the concentration-dependent toxicity of surfactants (e.g., polysorbates) used in drug formulations.28
ATCC’s Caco-2 cells are used in a variety of research fields but prove to be the gold standard for intestinal toxicology studies due to their ability to differentiate and form brush border microvilli. With so much potential, current research avenues with this cell line include drug transport studies utilizing microfluidic barrier model systems29 and toxicology studies of food-packaging material in relation to human health effects.30
Item 22/100: Neuroblast Workhorse
Ana Eckert, BS, Lead Innovation Specialist
ATCC® CCL-131™ – Neuro-2a
In May 1969, Dr. Robert Klebe and Dr. Frank Ruddle submitted Neuro-2a cells to ATCC. These cells are neuroblasts that were isolated from an albino mouse. These cells are useful in neuroscience and toxicology research due to their ability to reliably differentiate into neurons within just a few days. Because of this, they are used to study neuronal differentiation, axonal growth, and signaling pathways.31 Though their toxin responses can differ from intact neuron primary cells, they are still heavily used in toxicology research. Due to their ability to synthesize large amounts of microtubules, Neuro-2a cells have been used to study neurotoxicity, Alzheimer’s disease, Parkinson’s disease, neurite outgrowth, herpes simplex virus, poliovirus, and more.32 The World Organization for Animal Health (OIE) also uses Neuro-2a cells for the routine diagnosis of rabies. By leveraging these cells, researchers can advance their understanding of neurobiology and toxicology, contributing to safer and more effective treatments for various diseases.
Item 23/100: Dermal Fibroblast
Nicholas Lee, BS, Biologist
ATCC® CRL-4066™ – hTERT HDFa
Dermal fibroblasts play a crucial role in skin biology; these cells synthesize the extracellular matrix to generate and maintain connective tissue and they facilitate skin regeneration.33 While primary dermal fibroblasts have been widely used in skin disease, skin aging, wound healing, cosmetic, and toxicology studies34-37 cellular senescence and donor availability have restricted their potential usefulness. Immortalized cells can overcome the restrictions of primary cells and can be used as an alternative model to primary dermal fibroblasts.38,39 To meet this need, ATCC developed hTERT HDFa—a dermal fibroblast cell line that has been immortalized by stably expressing hTERT and CDK4R24C genes in primary dermal fibroblast cells. This immortalized cell line retains functional characteristics of primary cells and can be used as an in vitro cell model for skin disease, skin aging, wound healing, cosmetic, and toxicology studies.
Item 24/100: Spheroids and 3-D Cell Culture Models
Carolina Lucchesi, PhD, Principal Scientist
ATCC® SCM-CCL-247-2PLT™ – ThawReady™ HCT 116 Spheroid Kit
3-D cell culture models are revolutionizing the field of oncology by providing more accurate and reliable data compared to traditional 2-D cultures. These advanced models, such as spheroids, organoids, and scaffold-based systems, mimic the complex architecture and microenvironment of human tissues more closely.40 ATCC offers a range of 3-D cell culture models to support the scientific community. These models include patient-derived cancer organoids and Thaw-Ready™ Spheroids, which are essential for advancing research in oncology and other biomedical fields. ATCC’s ThawReady™ technology provides a simple, convenient method for generating spheroids for high-throughput workflows. 3-D models can be derived from human stem cells or patient-specific cells, offering personalized insights into cancer biology and treatment responses. This is particularly valuable in identifying individual variations in drug responses and potential adverse effects. By closely mimicking the human tissue environment, these models help in understanding the mechanisms of cancer progression at a cellular level, which is crucial for developing more effective and targeted therapies.41
Did you know?
ATCC’s vast portfolio of cells, media, and reagents support each step of the in vitro preclinical testing process—from modeling, screening, and characterization to exploratory toxicology, pharmacokinetics, and metabolism.
Explore more resources
100 for 100: A Centennial Celebration of the ATCC Collection
In this first post in our 100 for 100 series, learn about the microorganisms that are special to our staff.
More100 for 100: Looking back at ATCC’s most influential deposits
In this second post in our 100 for 100 series, learn about our most influential cell lines and microorganisms.
More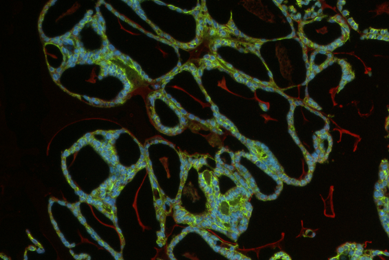
Toxicology and Safety Testing
Streamline your research with advanced cell models that support every stage of your toxicological screening.
MoreReferences
- Lazarus JV, et al. Advancing the global public health agenda for NAFLD: a consensus statement. Nat Rev Gastroenterol Hepatol 19(1): 60–78, 2022. PubMed: 34707258
- Sivell C. Nonalcoholic Fatty Liver Disease: A Silent Epidemic. Gastroenterol Nurs 42(5): 428-434, 2019. PubMed: 31574071
- Dworken HJ. CHAPTER 7 - The Liver: Structure and Function. In: Gastroenterology. Butterworth-Heinemann; 1982: 173-199.
- Zeilinger K, et al. Cell sources for in vitro human liver cell culture models.Exp Biol Med (Maywood) 241(15): 1684-1698, 2016. PubMed: 27385595
- Beghini DG, et al. Induced Pluripotent Stem Cells in Drug Discovery and Neurodegenerative Disease Modelling.Int J Mol Sci 25(4): 2392, 2024. PubMed: 38397069
- Ghosheh M, et al. Electro-metabolic coupling in multi-chambered vascularized human cardiac organoids. Nat Biomed Eng 7(11): 1493–1513, 2023. PubMed: 37550423
- Sharma A, et al. Human iPSC-Derived Cardiomyocytes as an Effective Preclinical Model for Drug Evaluation. J Pharmacol Toxicol Methods, 102: 106676, 2020.
- Lopez-Suarez L, et al. The SH-SY5Y human neuroblastoma cell line, a relevant in vitro cell model for investigating neurotoxicology in human: Focus on organic pollutants. Neurotoxicology. 92: 131-155, 2022. PubMed: 35914637
- SH-SY5Y: Human neuroblastoma cell line (ATCC CRL-2266). Memorial Sloan Kettering Cancer Center.
- Ciccarone V, et al. Phenotypic diversification in human neuroblastoma cells: expression of distinct neural crest lineages. Cancer Res 49(1): 219-225, 1989. PubMed: 2535691
- Filograna R, et al. Analysis of the Catecholaminergic Phenotype in Human SH-SY5Y and BE(2)-M17 Neuroblastoma Cell Lines upon Differentiation. PLoS ONE 10(8): e0136769, 2015. PubMed: 26317353
- Kovalevich J, Langford D. Considerations for the use of SH-SY5Y neuroblastoma cells in neurobiology. Methods Mol Biol 1078: 9-21, 2013. PubMed: 23975817
- Encinas M, et al. Sequential treatment of SH-SY5Y cells with retinoic acid and brain-derived neurotrophic factor gives rise to fully differentiated, neurotrophic factor-dependent, human neuron-like cells. J Neurochem 75(3): 991-1003, 2000. PubMed: 10936180
- Forster JI, et al. Characterization of Differentiated SH-SY5Y as Neuronal Screening Model Reveals Increased Oxidative Vulnerability. J Biomol Screen 21(5): 496-509, 2016. PubMed: 26738520
- Khazeem MM, et al. TOP2B Is Required to Maintain the Adrenergic Neural Phenotype and for ATRA-Induced Differentiation of SH-SY5Y Neuroblastoma Cells. Mol Neurobiol 59(10): 5987-6008, 2022. PubMed: 35831557
- Niaz A, et al. Robust Dopaminergic Differentiation and Enhanced LPS-Induced Neuroinflammatory Response in Serum-Deprived Human SH-SY5Y Cells: Implication for Parkinson's Disease. J Mol Neurosci 71(3): 565-582, 2021. PubMed: 32789724
- Martin ER, Gandawijaya J, Oguro-Ando A. A novel method for generating glutamatergic SH-SY5Y neuron-like cells utilizing B-27 supplement. Front Pharmacol 13: 943627, 2022. PubMed: 36339621
- D'Aloia A, et al. A new advanced cellular model of functional cholinergic-like neurons developed by reprogramming the human SH-SY5Y neuroblastoma cell line. Cell Death Discov 10(1): 24, 2024. PubMed: 38216593
- Zabner J, et al. Development of cystic fibrosis and noncystic fibrosis airway cell lines. Am J Physiol Lung Cell Mol Physiol 284(5): L844–L854, 2003. PubMed: 12676769
- Bani Melhim S, et al. The effect of triple CFTR modulator therapy and azithromycin on ion channels and inflammation in cystic fibrosis. ERJ Open Res 10(6): 00502-2024, 2024. PubMed: 39687397
- Jarosz-Griffiths HH, et al. Heightened mitochondrial respiration in CF cells is normalised by triple CFTR modulator therapy through mechanisms involving calcium. Heliyon 10(20): e39244, 2024. PubMed: 39498005
- Gwatimba A, et al. AI-Driven Cell Tracking to Enable High-Throughput Drug Screening Targeting Airway Epithelial Repair for Children with Asthma. J Pers Med 12(5): 809, 2022. PubMed: 35629232
- Fogh J, Wright WC, Loveless JD. Absence of HeLa cell contamination in 169 cell lines derived from human tumors. J Natl Cancer Inst 58(2): 209-214, 1977. PubMed: 833871
- Hidalgo IJ, Raub TJ, Borchardt RT. Characterization of the human colon carcinoma cell line (Caco-2) as a model system for intestinal epithelial permeability. Gastroenterology 96(3): 736-749, 1989. PubMed: 2914637
- Zucco F, De Angelis I, Testai E, Stammati A. Toxicology investigations with cell culture systems: 20 years after. Toxicol In Vitro 18(2):153-163, 2004. PubMed: 14757105
- Kus M, Ibragimow I, Piotrowska-Kempisty H. Caco-2 Cell Line Standardization with Pharmaceutical Requirements and In Vitro Model Suitability for Permeability Assays. Pharmaceutics 15(11): 2523, 2003. PubMed: 38004503
- Ghasemi M, et al. The MTT Assay: Utility, Limitations, Pitfalls, and Interpretation in Bulk and Single-Cell Analysis. Int J Mol Sci 22(23): 12827, 2021. PubMed: 34884632
-
Ujhelyi Z, et al. Evaluation of cytotoxicity of surfactants used in self-micro emulsifying drug delivery systems and their effects on paracellular transport in Caco-2 cell monolayer. Eur J Pharm Sci 47(3): 564-573, 2012. PubMed: 22841998
- Tan HY, et al. A multi-chamber microfluidic intestinal barrier model using Caco-2 cells for drug transport studies. PLoS One 13(5): e0197101, 2018. PubMed: 29746551
- Cebadero-Domínguez O, et al. In vitro toxicity evaluation of graphene oxide and reduced graphene oxide on Caco-2 cells. Toxicol Rep 9: 1130-1138, 2022. PubMed: 36518447
- Tremblay RG, et al. Differentiation of mouse Neuro 2A cells into dopamine neurons. J Neurosci Methods 186(1): 60–67, 2010. PubMed: 19903493
- LePage KT, et al. On the use of neuro-2a neuroblastoma cells versus intact neurons in primary culture for neurotoxicity studies.Crit Rev Neurobiol 17(1): 27-50, 2005. PubMed: 16307526
- Plikus MV, et al. Fibroblasts: origins, definitions, and functions in health and disease. Cell 184(15): 3852-3872, 2021. PubMed: 34297930
- Zorina A, et al. Dermal Fibroblasts as the Main Target for Skin Anti-Age Correction Using a Combination of Regenerative Medicine Methods. Curr Issues Mol Biol 45(5): 3829-3847, 2023. PubMed: 37232716
- Stunova A, et al. Dermal fibroblasts-A heterogeneous population with regulatory function in wound healing. Cytokine Growth Factor Rev 39: 137-150, 2018. PubMed: 29395658
- Groeber F, et al. Skin tissue engineering--in vivo and in vitro applications. Adv Drug Deliv Rev 63(4-5): 352-66, 2011. PubMed: 21241756
- Rahnama M, et al. A comprehensive evaluation of dermal fibroblast therapy in clinical trials for treating skin disorders and cosmetic applications: a scoping review. Stem Cell Res Ther 15(1): 318, 2024. PubMed: 39304949
- Bodnar AG, et al. Extension of Life-Span by Introduction of Telomerase into Normal Human Cells. Science 279(5349): 349-52, 1998. PubMed: 9454332
- Voloshin N, et al. Practical Use of Immortalized Cells in Medicine: Current Advances and Future Perspectives. Int J Mol Sci 2023 Aug 12;24(16):12716. PubMed: 37628897
- Poornima K, et al. Implications of Three-Dimensional Cell Culture in Cancer Therapeutic Research.Front Oncol 12: 891673, 2022. PubMed: 35646714
- Abuwatfa WH, et al. Scaffold-based 3D Cell Culture Models in Cancer Research. J Biomed Sci 31(1): 7, 2024. PubMed: 38221607