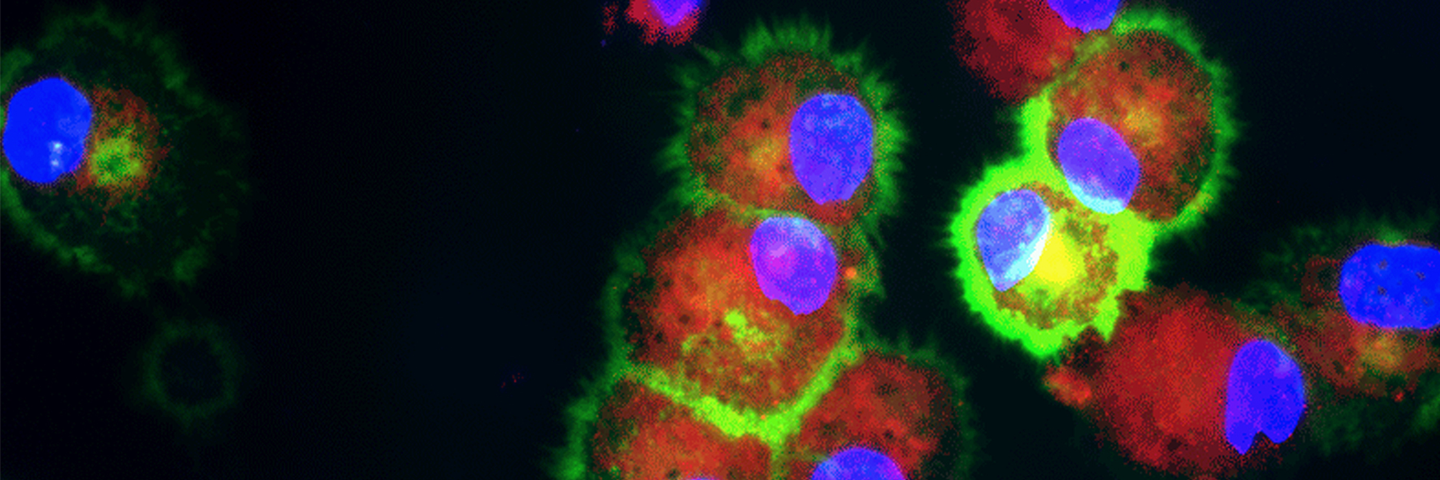
Authors: James Clinton, PhD and Brian Shapiro, PhD
This study will demonstrate the differentiation potential of cryopreserved primary human CD14+ monocytes towards macrophage and dendritic cell lineages for applications such as cancer immunology, vaccine development, inflammation, bone disease, immunosuppression, disease resolution, and tissue repair studies.
Figure 1. Schematic depicting in vivo monocyte differentiation and downstream functions.
Monocytes are large, mononuclear leukocytes that are generated in bone marrow from myeloblast progenitors. After an initial period of differentiation, these cells enter peripheral blood circulation and eventually migrate into tissue. There, monocytes mature and serve important functions in the innate and adaptive immune systems, particularly in their role as precursor cells to macrophages and dendritic cells.1 Monocytes replenish tissue-specific macrophage populations such as osteoclasts in bone, microglia in the CNS, and Kupffer cells in the liver. In addition to contributing to an animal’s defense against microorganisms, macrophages also have an important role in the removal of cellular debris as well as dead and senescing cells. Dendritic cells are antigen-presenting cells that function as part of the adaptive immune system by endocytosing, processing, and then presenting antigens to stimulate T-cell immune activity. Monocytes are a heterogeneous population consisting of several subtypes, but are primarily defined by CD14+ expression.2 These subsets exhibit diverse phenotypes, showing differential responses to infection, phagocytic activity, and cytokine production under various conditions. In vivo, monocytes respond to activating stimuli such as cytokines and bacterial peptides to initiate differentiation (Figure 1). In vitro, similar signals may be utilized to selectively induce differentiation into either macrophages or dendritic cells. These monocyte-derived cells exhibit distinct phenotypes including altered morphology and marker expression. The ability to easily and quickly generate large numbers of human-derived macrophages and dendritic cells ex vivo allows for an alternative to the use of classic monocytic cell lines which, while commonly induced to exhibit a differentiated-like state using various methods such as phorbol-12-myristate-13-acetate treatment,3-5 may not fully recapitulate the human primary cell phenotype and may result in unwanted gene activation.6,7 Here, we demonstrate that with two simple and quick protocols, cryopreserved primary human CD14+ monocytes (ATCC PSC-800-010) can be differentiated into macrophages and dendritic cells suitable for a wide variety of downstream applications.
Download a PDF of this application note
Download NowMaterials and methods
For macrophage differentiation, CD14+ monocytes were seeded in ultra-low attachment plates (Corning) at 1 × 106/cm2 in Alpha Minimum Essential Medium with Nucleosides (Sigma-Aldrich) supplemented with 10% Fetal Bovine Serum (FBS; ATCC 30-2020) and 500 U/mL granulocyte macrophage-colony stimulating factor (GM-CSF; Peprotech). After incubating for 1.5 h in a 5% CO2 incubator at 37°C, the plates were swirled vigorously to remove loosely attached cells. The plates were then washed three times with warm media and returned to the incubator. Complete media change was performed every 3 days. After 9 days, the adherent cells were collected for analysis. To harvest cells without enzymatic digestion, the cells were incubated in ice cold 2 mM EDTA in Dulbecco’s Phosphate Buffered Saline (ATCC 30-2200) with the plate kept on ice for 15 minutes. Cells were collected by repeated pipetting. For dendritic cell differentiation, CD14+ monocytes were seeded at 0.5 × 106/cm in RPMI-1640 (ATCC 30-2001) containing 10% FBS. Media was changed after 24 hours, and then every 3 days. During media changes, the supernatant was retained, pelleted at 300 x g for 5 minutes, and the collected cells returned to the wells. Maturation factors, including 1000 U/mL GM-CSF and 500 U/mL interleukin-4 (IL-4; Peprotech), were added to the media starting on day 6. After an additional 3 days, the non-adherent cells were collected for analysis. Viability was determined using a Vi-CELL Cell Counter (Beckman Coulter). Marker expression was measured using flow cytometry with a BD Accuri C6 flow cytometer (BD Biosciences). Immunofluorescence expression analysis was performed with fluoresceinisothiocyanate (FITC)- or phycoerythrin (PE)-labeled mouse anti-human CD14, CD45, CD68, CD80, CD83, and CD163, and FITC- or PE-conjugated, isotype-matched antibodies (BD Pharmingen) were used as controls. Five to ten thousand events were collected per sample.
Results and discussion
After 9 days in macrophage differentiation media, the CD14+ monocytes exhibited the morphological characteristics typical of type 1 macrophages (Figure 2), with viability over 85%. Marker expression was also consistent with type 1 macrophages, with high expression of CD68 and CD80, and reduced expression of CD14 (Figure 3). Low expression of CD163 indicated that minimal type 2 macrophages were produced. An alternate protocol utilizing M-CSF instead of GM-CSF would likely bias generation towards type 2 macrophages; however, this was not tested. The addition of activating factors such as interferon-γ, lypopolysaccharide, or tumor necrosis factor-α could be utilized to further polarize the macrophages.
After 24 hours in dendritic cell differentiation media, the cells displayed a heterogeneous mix of floating and adherent cells (Figure 4). After 6 days in media, the majority of CD14+ monocytes exhibited morphological characteristics typical of immature dendritic cells, with the majority of the cells non-adherent (Figure 4). Some undifferentiated cell clusters remained at this stage. After an additional 3 days (9 days total) in differentiation media, all the cells displayed the characteristic mature phenotype (Figure 4). Marker expression revealed high expression of CD80 and CD83, and negligible expression of CD14, a phenotype consistent with mature dendritic cells (Figure 5).
Figure 2. Monocyte-derived macrophage morphology at A) low power and B) high power. Phase contrast microscopy indicates that at day 9, post-differentiation cells exhibit typical type M1 macrophage morphology. Scale bars are A) 1000 μm (4X) and B) 100 μm (40X).
Figure 3. Monocyte-derived macrophage morphology marker expression. Nine days after differentiation, monocyte-derived macrophages exhibited high expression of CD68 and CD80, reduced expression of CD14, and minimal expression of CD163. N = 3, error bars represent the standard deviation of the mean (STD).
Figure 4. Monocyte-derived dendritic cell morphology at A) day 1, B) day 6, and C) day 9. B) Phase contrast micrographs show that by day 6 floating immature dendritic “veiled” cells are visible. C) After another 3 days in differentiation media, the cells in suspension consist of mature dendritic cells as indicated by the sharp processes. Scale bars are A) 1000 μm (4X), B and C) 100 μm (40X).
Figure 5. Monocyte-derived dendritic cell marker expression. Nine days post-differentiation, monocyte-derived dendritic cells exhibited high expression of CD80 and CD83 and minimal expression of CD14. N = 3, error bars represent STD.
Conclusion
ATCC primary human CD14+ monocytes are capable of differentiating into macrophages and dendritic cells as assessed by common surface markers and typical morphological characteristics. Generating primary human monocyte-derived macrophages and dendritic cells may be a suitable alternative to monocytic cell lines in applications where phenotypical relevance is of critical concern, or as a control for novel immune cell models.
Download a PDF of this application note
Download NowProducts used in this research
References
1. Serbina N, et al. Monocyte-mediated defense against microbial pathogens. An Rev Immunol 26:421, 2008.
2. Passlick B, Flieger D, Ziegler-Heitbrock HW. Identification and characterization of a novel monocyte subpopulation in human peripheral blood. Blood 74:2527–34, 1989.
3. Tsuchiya S, et al. Induction of maturation in cultured human monocytic leukemia cells by a phorbol diester. Canc Res 42(4):1530-1536, 1982.
4. Cassileth P, Suholet D, Cooper R. Early changes in phosphatidylcholine metabolism in human acute promyelocytic leukemia cells stimulated to differentiate by phorbol ester. Blood 58(2):237, 1981.
5. Liu M, Wu M. Induction of human monocyte cell line U937 differentiation and CSF-1 production by phorbol ester. Exp Hema 20(8):974-979, 1992.
6. Daigneault M, et al. The identification of markers of macrophage differentiation in PMA-stimulated THP-1 cells and monocyte-derived macrophages. PloS one 5(1): e8668, 2010.
7. Kohro T, et al. A comparison of differences in the gene expression profiles of phorbol 12-myristate 13-acetate differentiated THP-1 cells and human monocyte-derived macrophage. J Athero Thromb 11(2):88-97, 2004.