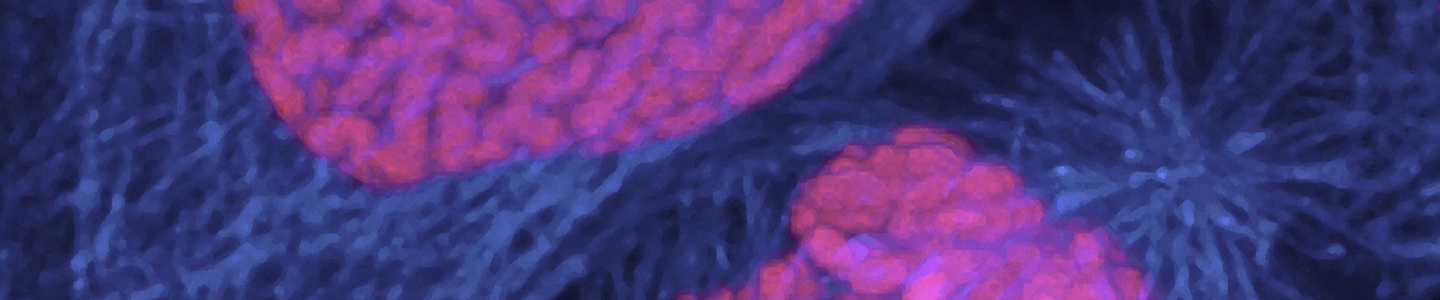
By Fang Tian, PhD, and Elizabeth Turner, PhD
Researchers and clinicians have been waging a war on cancer for over five decades, and significant progress has been achieved. However, despite an increase in the number of therapeutics available, cancer is still the second most common cause of death; the number of deaths from cancer in 2019 is predicted to be about 606,880 in the United States alone.1 Statistical data indicate that over 90% of the mortality of patients with cancer is related to drug resistance.2 Thus, the persistence of drug resistance remains a major challenge in this field. This constitutes a serious concern for patients as resistance to therapeutics can lead to decreased drug response and the recurrence of disease. While efforts are being made to identify the mechanisms of resistance and to develop novel drugs and therapeutic agents to overcome them, the lack of physiologically relevant, drug-resistant cancer model systems remains a significant challenge.
Drug resistance in cancer comes in two forms: intrinsic resistance and acquired resistance. Intrinsic resistance is present before a therapy is administered, and it results in the inherent ability of cancer cells to survive at clinically relevant concentrations of a drug. A poor initial response to the given treatment indicates that a patient may have intrinsic drug resistance. Conversely, acquired drug resistance occurs after an initial positive response to the therapy. In this case, the cancer cells gradually acquire specific genetic abnormalities or protein alterations that ultimately result in unresponsiveness to treatment and cancer relapse.3 Acquired resistance to an initially effective therapy is a much greater challenge in the clinical setting as optimism following initial treatment and response can quickly turn into poor results and devastating outcomes. The phenomenon of acquired drug resistance has been observed with various types of cancer therapeutics including chemotherapy, molecular-targeted anticancer drugs, and cancer immunotherapy.4,5 Precision medicine approaches, such as liquid biopsy, allow for monitoring the patient’s response to a treatment in real time. Although this brings a hope to identify drug resistance and detect the cancer’s relapse at an early stage, the corresponding treatment solutions are not always available.
The overall cause of drug resistance is complex, and several categories of drug-resistance mechanisms have been identified. The major mechanisms of resistance include tumor evolution and tumor heterogeneity, acquired secondary genetic alterations, signaling pathway feedback loops and bypass mechanisms, and the tumor microenvironment.4,5 Drug resistance in each case could involve one or more of these major mechanisms, depending on the class of drugs and therapeutic treatment strategies being employed.5,6 Cancer cells are highly plastic and adaptable, evolving and accumulating irreversible genetic alterations throughout the initiation and development of the tumor.7 The genetic abnormalities and drug sensitivities of cells within the same tumor can vary widely, resulting in the survival and regrowth of a sub-population of tumor cells after a treatment that initially appears to be effective.3,4 In some cases, tumor cells can even acquire secondary genetic alterations during drug treatment. These mutations can give the cell the ability to withstand further treatment with that drug. In addition to acquiring somatic mutations associated with drug resistance, cancer cells may alter cell signaling pathway feedback loops or bypass certain drug target signaling pathways through epigenetic alterations to maintain activation of key cell proliferation and survival signals. Furthermore, tumors cells can induce surrounding normal tissue cells to alter the overall microenvironment by secreting growth factors or proteases, or by suppressing immune response signals. Although our knowledge of some individual drug-resistance mechanisms has expanded significantly, a comprehensive understanding and means of addressing multifactorial drug-resistance mechanisms has yet to be achieved.
Resources for drug-resistance research
CRISPR/Cas9-engineered Cancer Models
Learn about the use of CRISPR/Cas9 in engineering cell models that mimic specific cancer genotypes and phenotypes found in clinical tumor samples.
Cancer research
Surviving cancer relies on an early, accurate diagnosis of the disease combined with effective treatment. That's why ATCC provides the cell models and nucleic acids needed to develop and validate diagnostic assays.
Cell-based models are critical tools for understanding the mechanisms of drug resistance and developing novel therapeutics. The most common method for generating a drug-resistant cancer cell line involves repeated treatment of the cells with clinically relevant anticancer drugs for an extended period of time. Dosage is often gradually increased during the culture selection process, so the development of a drug-resistant cell line can take 6-12 months or longer.8 Although many laboratories have been working to derive new cancer cell lines, there remains a lack of drug-resistant in vitro models. To address this need, ATCC has collected more than 50 well-established and characterized drug-resistant cell lines to facilitate cancer research. This growing portfolio includes cell lines resistant to commonly used chemotherapy drugs such as doxorubicin, specific targeted cancer therapies such as the kinase inhibitor trastuzumab (Herceptin), and estrogen modulators such as tamoxifen. Several of the unique tumor cell lines express multidrug resistance gene MDR1 and are significantly resistant to multiple anticancer drugs.
To improve on the traditional model of drug resistance, ATCC has been developing state-of-the-art drug-resistant cancer cell models by using CRISPR-Cas9 gene-editing technology to introduce critical mutations into disease-relevant cell lines. These novel cell lines overcome several drawbacks of drug-resistant cancer models that have been developed using the traditional progressive drug selection method. These shortcomings include cell line heterogeneity, instability of the relevant genotype, the requirement for continued drug pressure to maintain the cell line, and the lack of models for acquired resistance to newly developed therapeutics. A notable example of acquired drug resistance is the development of resistance to BRAF inhibitor therapy in melanoma patients. ATCC scientists used CRISPR-Cas9 gene-editing technology to introduce the specific point mutations associated with acquired BRAF inhibitor resistance directly into A375 human malignant melanoma cells and developed KRASG13D A375 (CRL-1619IG-1), NRASQ61K A375 (CRL-1619IG-2), and MEK1Q56P A375 (CRL-1619IG-3) Isogenic Cell Lines. These new drug-resistant cell lines are powerful new tools for evaluating secondary mutations associated with BRAF-inhibitor resistance in the absence of any selective drug pressure under physiologically relevant conditions. Furthermore, because these mutations have been precisely engineered using CRISPR-Cas9 rather than by selective drug pressure, each new engineered cell line is genetically identical to the cell line from which it was derived, except for the introduced point mutation. Thus, each engineered model cell line can be used together with its parental line as an isogenic pair for mechanistic studies or for screening new therapeutics that can address the modeled drug resistance.
Strategies for preventing and overcoming drug resistance in cancer treatment remain limited. The complexity of cancer genomics and tumor biology allows a tumor to evolve new resistance mechanisms to seemingly any available or emerging cancer therapeutics. Nevertheless, scientists and physicians have been focusing on a combination approach to cancer therapy in recent years. This combination therapy strategy works by targeting multiple key molecules in the same signaling pathway, multiple pathways in the same tumor, or targeting both cancer cells and immune cells. Using this approach, clinical outcomes in drug resistant cancer patients can be improved by employing multiple treatment modalities such as chemotherapy, immunotherapy, and targeted-therapy regimens in various combinations.5,9 The overall effectiveness of combination therapy is still under investigation, and the number of proposed combination treatment plans is enormous. Evaluation of new combination cancer therapies is currently done via costly, low-throughput animal studies and clinical trials. There is therefore an urgent need for complex, three-dimensional and multicomponent in vitro cancer models that mimic the plasticity of tumor cells and the tumor microenvironment. This next generation of advanced cancer models are vital tools for furthering our understanding drug-resistance mechanisms and will help lead to faster development cycles and lower failure rates for both anticancer drug development and evaluation of combination therapy strategies.
Download a PDF of this white paper
Download NowReferences
- Cancer Facts & Figures 2019. American Cancer Society
- Si W, et al. The role and mechanisms of action of microRNAs in cancer drug resistance. Clin Epigenetics. 11(1):25, 2019.
- Chatterjee N, et al. Polytherapy and targeted cancer drug resistance. Trends Cancer 5(3):170-182, 2019.
- McGranahan N, et al. Clonal heterogeneity and tumor evolution: Past, present, and the future. Cell 168(4):613-628, 2017.
- Konieczkowski DJ, et al. A convergence-based framework for cancer drug resistance. Cancer Cell 33(5):801-815, 2018.
- Gupta PB, et al. Phenotypic plasticity: Driver of cancer initiation, progression, and therapy resistance. Cell Stem Cell 24(1):65-78, 2019.
- Housman G, et al. Drug resistance in cancer: An overview. Cancer 6(3): 1769-1792, 2014
- McDermott M, et al. In vitro development of chemotherapy and targeted therapy drug-resistant cancer cell lines: A practical guide with case studies. Front Oncol 4:40, 2014.
- Patel S, et al. Combination cancer therapy with immune checkpoint blockade: Mechanisms and strategies. Immunity 48(3):417-433, 2018.