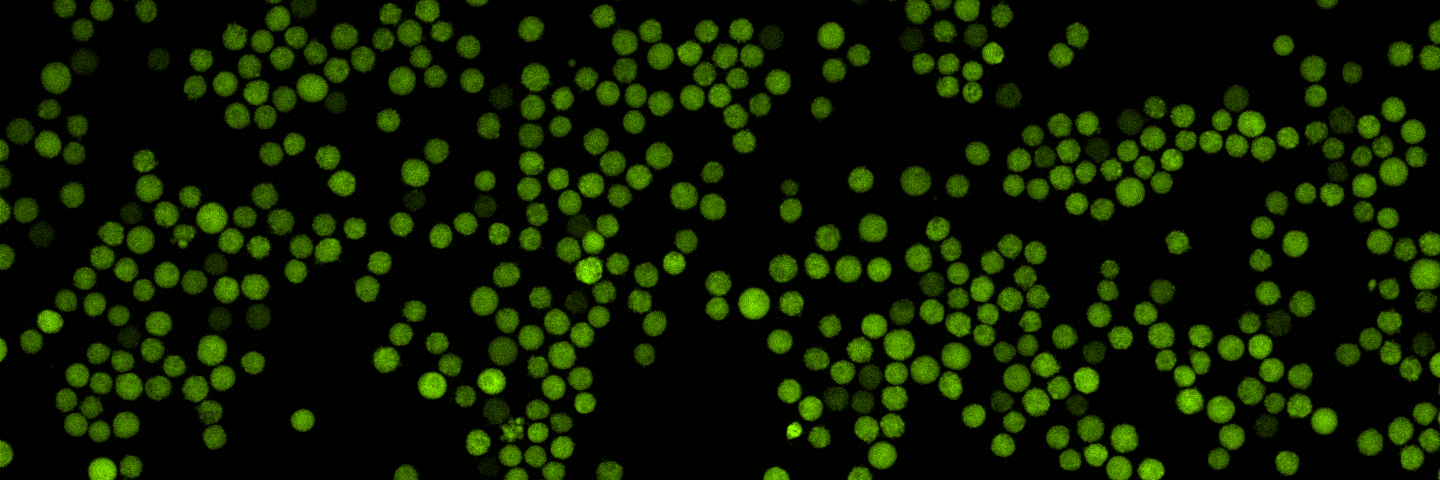
Authors: James Clinton, PhD; and Brian Shapiro, PhD
Introduction
Methods
Results and Discussion
Conclusion
Figures
Abstract
This study will examine the differentiation and expansion potential of bone marrow-derived (BM) CD34+ cells into erythroid cells, megakaryocytes, and myeloid precursor cells in a liquid, non-adherent culture format.
Download a PDF of this application note
Download NowIntroduction
Hematopoiesis, or the replacement of blood cells, is critical for the normal functions of the cardiovascular and immune systems. In hematopoiesis, hematopoietic stem and progenitor cells (HSPCs) may differentiate down several lineages to form red blood cells, platelets, or leukocytes. CD34 is a molecular marker of undifferentiated HSPCs; its absence suggests that the cells have progressed down one of the various hematopoietic cell lineages.1 Additionally, as they differentiate, the cells express various markers that are commonly used to indicate lineage: GlyA and CD71 for erythroid (red blood cell precursor) progenitor cells,2 CD41a and CD42b for megakaryocytes (platelet precursor),3 and CD33 and CD11b for myeloid (granulocyte and monocyte precursor) progenitor cells.4,5 Varying the hormone and cytokines components in vitro induces differentiation in BM CD34+ cells along the various hematopoietic lineages.6 Here, we describe protocols for the expansion and differentiation of BM CD34+ cells in suspension culture into erythroid, megakaryocyte, and myeloid precursors.
Methods
Primary BM CD34+ cells (ATCC PCS-800-012) were isolated and purified using positive immunomagnetic selection, then cryopreserved in CryoStor CS10 Freeze Medium (BioLife Solutions). After rapidly thawing the vial at 37°C, cells were transferred to ice cold Hank’s Balanced Salt Solution (ATCC 30-2213) supplemented with 10% Fetal Bovine Serum (ATCC 30-2020). The percentage of CD34+ cells was greater than 95% as measured by flow cytometry. The cells were then centrifuged at 4°C at 300 x g for 5 minutes to pellet, then re-suspended in the appropriate differentiation media and seeded into low attachment multiwell plates.
For erythroid differentiation, BM CD34+ cells in erythroid expansion media [StemSpan SFEM II (StemCell Technologies) supplemented with the StemSpan Erythroid Expansion Supplement (StemCell Technologies)] were seeded at 5x103 cells/mL in 1 mL per well in a 24-well plate. Forty-eight hours later, the cells were gently mixed and 500 μL fresh erythroid expansion media was added to the wells. Seventy-two hours later, the cells were gently mixed and 1 mL fresh erythroid expansion media was added to the wells. Forty-eight hours later, the cells were collected and analyzed for expression of the erythroid lineage markers GlyA and CD71.
For megakaryocyte differentiation, BM CD34+ cells in megakaryocyte expansion media [SFEM II plus StemSpan Megakaryocyte Expansion Supplement (StemCell Technologies)] were seeded at 1x104 cells/mL in 1 mL per well in a 12-well plate. Every days, 500 μL of fresh megakaryocyte expansion media was added. The cells were pelleted and re-suspended in fresh megakaryocyte expansion media every 7 days. On days 14 and 28, the cells were analyzed for the expression of the megakaryocyte lineage markers CD41a and CD42b.
For myeloid differentiation, BM CD34+ cells in myeloid expansion medium [SFEM II supplemented with StemSpan CC100 Cocktail (StemCell Technologies) plus 20 ng/mL granulocyte macrophage-colony stimulating factor (GM-CSF; Biolegend)] were seeded at 1x104 cells/mL in 1 mL per well in a 12-well plate. Every 2 days, 500 μL of fresh myeloid expansion media was added. The cells were pelleted and re-suspended in fresh myeloid expansion media every 7 days. The cells were analyzed on days 7 and 14 for the expression of the myeloid lineage markers CD33 and CD11b.
Total cell numbers and viability were determined using a Vi-CELL Cell Counter (Beckman Coulter). Marker expression was measured using flow cytometry with a BD Accuri C6 flow cytometer (BD Biosciences). Immunofluorescence expression analysis was performed with fluorescein isothiocyanate (FITC)-labeled mouse anti-human CD34, CD41a, CD33, and CD71 (BD Pharmingen), or with phycoerythrin (PE)-labeled mouse anti-human CD11b, CD42b, and GlyA (BD Pharmingen). FITC or PE conjugated, isotype-matched antibodies (BD Pharmingen) were used as controls. Five to ten thousand events were collected per sample.
Results and Discussion
BM CD34+ cells cultured for 7 days in medium containing StemSpan Erythroid Expansion Supplement exhibited 550-fold expansion of total nucleated cells (TNC) with high viability (Figure 1). In addition, greater than 90% of cells were positive for erythroid lineage markers, while less than 20% of cells expressed CD34 (Figure 2). The GlyA+CD71+ cells constitute mid-stage erythroblasts. Additional factors would be required during culture to continue the progression of differentiation in these cells, eventually resulting in the expulsion of the nucleus and the subsequent transition into mature erythrocytes.
BM CD34+ cells cultured for 14 days in medium containing StemSpan Megakaryocyte Expansion Supplement displayed 66-fold expansion of TNC with high viability (Figure 1). On day 14, over 90% of cells were positive for megakaryocyte lineage markers, while fewer than 5% of cells expressed CD34 (Figure 3). The population of mature CD41a+CD42b+ megakaryocytes rapidly increased during the initial 14 days of differentiation and then slowed between days 14 and 28. Some immature promegakaryocytes (CD41a+CD42b-) remained at day 28.
BM CD34+ cells cultured for 14 days in medium containing StemSpan CC100 Cocktail and GM-CSF showed greater than 1000-fold expansion of TNC with high viability (Figure 1). On day 14, 70% of cells were positive for a pan-myeloid marker with less than 5% of cells expressing CD34 (Figure 4 A-C). The CD11b+ fraction likely contains a mixed population of macrophages, monocytes, and granulocytes. Interestingly, the percentage of CD33+ cells remained largely unchanged from post-thaw (Figure 4 B-D). The initial CD34+CD33+ population likely represented mid-stage myeloid committed progenitors (myeloblasts). Post-differentiation CD33 expression may reflect the presence of various immature myeloid cells including monocytes and granulocytes. Confirming myeloid lineage-specific expansion, the percentage of cells expressing markers for erythrocytes and megakaryocytes was found to be less than 10% (Figure 5).
Conclusion
When grown in the appropriate culture conditions, ATCC primary BM CD34+ hematopoietic cells expanded and differentiated into erythrocyte, megakaryocyte, and myeloid precursor cells with high specificity, efficiency, and viability. The ability to generate lineage-specific precursors in vitro enables the study of lineage-specific phenotypes such as differences in toxicity in response to myelosuppressive drugs.7-9 Further, the physiological relevance of hematopoietic progenitor cells generated in vitro has been previously demonstrated by the correlated drug effects from in vitro and in vivo models.10,11 Therefore, the cells created by the protocols described herein are applicable for numerous downstream purposes, including engraftment assays, gene therapy, cytokine production, and drug screening studies.
Figures
Figure 1. Viability of BM CD34+ cells pre- and post-differentiation. Viability was assessed via trypan blue exclusion before or after culture in the indicated differentiation media at the specified time points.
![]() |
![]() |
![]() |
Figure 2. Expression of erythroid markers before and after lineage specific differentiation. Flow cytometry scatter plots depicting expression of the HSPC marker CD34 and erythroid lineage markers GlyA and CD71 before (A) and after (B, C) 7 days in culture. The percentage of CD34+ cells decreased from over 95% at day 0 to <20% after 7 days; simultaneously, the expression of erythroid markers increased from <5% at day 0 to over 90% on day 7.
![]() |
![]() |
![]() |
![]() |
![]() |
![]() |
![]() |
![]() |
![]() |
![]() |
![]() |
![]() |
![]() |
![]() |
Download a PDF of this application note
Download NowReferences
- Srour EF, et al. Human CD34+ HLA-DR-bone marrow cells contain progenitor cells capable of self-renewal, multilineage differentiation, and long-term in vitro hematopoiesis. Blood Cells 17(2):287-295, 1990.
- Luck L, et al. Human CD34+ and CD34+CD38− hematopoietic progenitors in sickle cell disease differ phenotypically and functionally from normal and suggest distinct subpopulations that generate F cells. Exp Hematol 32(5):483-493, 2004.
- Kratz-Albers K, et al. Effective ex vivo generation of megakaryocytic cells from mobilized peripheral blood CD34+ cells with stem cell factor and promegapoietin. Exp Hematol 28(3):335-346, 2000.
- Yoo ES, et al. Myeloid differentiation of human cord blood CD34+ cells during ex vivo expansion using thrombopoietin, flt3-ligand and/or granulocyte-colony stimulating factor. British J Haematol 105(4):1034-1040, 1999.
- Bühring H, et al. Sequential expression of CD34 and CD33 antigens on myeloid colony-forming cells. Euro J Haematol 42(2):143-149, 1989.
- Huss R. Isolation of primary and immortalized CD34− hematopoietic and mesenchymal stem cells from various sources. Stem Cells 18(1):1-9, 2000.
- Du DL, et al. In vitro myelotoxicity of 2’, 3’-dideoxynucleosides on human hematopoietic progenitor cells. Exp Hematol 18(7):832-836, 1990.
- Du DL, et al. Effects of L-phenylalanine mustard and L-buthionine sulfoximine on murine and human hematopoietic progenitor cells in vitro. Can Res, 50(13):4038-4043, 1990.
- Scheding S., Nakeff A. Acute toxic effects of 3’-azido-3’-deoxythymidine (AZT) on normal and regenerating murine hematopoiesis. Exper Hematol 22(1):60-65, 1994.
- Volpe D, et al. Myelotoxic effects of the bifunctional alkylating agent bizelesin on human, canine and murine myeloid progenitor cells. Canc Ther Pharm 39(1-2):143-149, 1996.
- Parchment R, et al. (1994). In vivo-in vitro correlation of myelotoxicity of 9-methoxypyrazoloacridine [NSC-366140, PD115934] to myeloid and erythroid hematopoietic progenitors from human, murine, and canine marrow. J Natl Canc Inst 86(4): 273-280, 1994.